Application of processes stimulated by nonequilibrium plasma for large-tonnage decontamination of soils
DOI:
https://doi.org/10.15587/2706-5448.2023.274688Keywords:
electric discharge, water solution, electrohydraulic resonance soil decontamination, radionuclides, plasma cell, active particlesAbstract
The object of research is a new, potentially effective and practical process for the decontamination of radioactive soil, based on combination of plasma hydroseparation and plasma activation. The cleaning effect is ensured by the destruction of the bonds of radionuclides with soil particles due to a series of electrophysical discharges at which active particles and shock waves appear. In a designed setup, the process of plasma-chemical treatment is implemented in a plasma cell with a self-sustaining pulsating mode of burning an electric discharge, which occurs in an aqueous solution. The setup realizes a resonant increase in the intensity of shock waves, turbulence and multiple expansion of the core, such that the expansion of the plasma-liquid interface becomes a real basis for scaling up the setup. Regardless of the material of the electrodes and in a wide range of electrical conductivity (measured from 100 to 5,000 μS/cm), the restructuring of the combustion regime is accompanied by an increase in the size and stabilization of the luminous zone, fragmentation of bubbles, and an increase in the rate of their evacuation from the discharge zone. The main factors of such a restructuring are the channel dimensions and temperature of the solution. Various materials of the walls of plasma-chemical reactor have been tested: plexiglass, ceramics and stainless steel with the thickness of 2 mm. The maximum increase in the amplitude of resonance oscillations depends on the cell radius. A dynamic pressure, which in an individual discharge is about 5–15 mm of the water column at the mouth of the discharge, increases to 150–200 mm of the water column at the bottom of the plasma cell at resonance. An increase in efficiency is achieved by an optimal choice of the duration of the current phase and the distance between the electrodes, which is 15–30 mm. The voltage drop is 70–80 % across the spark discharge, the rest falls across the solution. The transition of the discharge to a periodic pulsating current mode with an increase in the temperature of the solution has been found. Tests on a mobile plasma-chemical facility for the process of plasma co-precipitation of radionuclides 137Cs, 134Cs and 90Sr with ferrocyanide sorbents under real conditions of hydroseparation of contaminated soil from fields around the Fukushima Daiichi have shown a decrease in organic substances in water by 40 times, and of radioactivity by 75 times.
Supporting Agency
- Presentation of research in the form of publication through financial support in the form of a grant from SUES (Support to Ukrainian Editorial Staff).
References
- Andriushin, I. A., Iudin, Iu. A. (2010). Obzor problem obrashcheniia s radioaktivnymi otkhodami i otrabotavshim iadernym toplivom. Saratov: FGUP «RFIaTc–VNIIEF», 119.
- Seida, V. A., Tcivun, A. P. (2012). Problemnye voprosy obrashcheniia s radioaktivnymi otkhodami na ChAES. Problemy Chernobylskoi zony otchuzhdeniia, 10, 40–53.
- Evrard, O., Laceby, J. P., Nakao, A. (2019). Effectiveness of landscape decontamination following the Fukushima nuclear accident: a review. SOIL, 5 (2), 333–350. doi: https://doi.org/10.5194/soil-5-333-2019
- Strategies and Practices in the Remediation of Radioactive Contamination in Agriculture (2016). Report of a Technical Workshop Vienna, 194.
- The Fukushima Daiichi accident (2015). Vienna: International Atomic Energy Agency, 5, 218.
- Greenpeace | Fukushima Daiichi 2011–2021 (2021). The decontamination myth and a decade of human rights violations, 47.
- Fujiwara, H., Kuramochi, H., Nomura, K., Maeseto, T., Osako, M. (2017). Behavior of radioactive cesium during incineration of radioactively contaminated wastes from decontamination activities in Fukushima. Journal of Environmental Radioactivity, 178–179, 290–296. doi: https://doi.org/10.1016/j.jenvrad.2017.08.014
- IAEA: Radioactive Waste Management Glossary (2003). Vienna: IAEA, 54.
- IAEA: Application of Thermal Technologies for Processing of Radioactive Waste (2006). IAEA TECDOC 1527, Vienna, 90.
- Decontamination guidelines (2013). Japanese Ministry of the Environment. Available at: http://josen.env.go.jp/en/policy_document/pdf/decontamination_guidelines_2nd.pdf Last accessed: 14.04.2019
- Japanese Ministry of the Environment: Webpage on Environmental Remediation. Available at: http://josen.env.go.jp/en/framework/pdf/basic_principles.pdf Last accessed: 14.04.2019
- Nakao, A., Ogasawara, S., Sano, O., Ito, T., Yanai, J. (2014). Radiocesium sorption in relation to clay mineralogy of paddy soils in Fukushima, Japan. Science of The Total Environment, 468-469, 523–529. doi: https://doi.org/10.1016/j.scitotenv.2013.08.062
- Nishikiori, T., Suzuki, S. (2017). Radiocesium decontamination of a riverside in Fukushima, Japan. Journal of Environmental Radioactivity, 177, 58–64. doi: https://doi.org/10.1016/j.jenvrad.2017.06.005
- Parajuli, D., Kitajima, A., Takahashi, A., Tanaka, H., Ogawa, H., Hakuta, Y. et al. (2016). Application of Prussian blue nanoparticles for the radioactive Cs decontamination in Fukushima region. Journal of Environmental Radioactivity, 151, 233–237. doi: https://doi.org/10.1016/j.jenvrad.2015.10.014
- Sakai, M., Gomi, T., Nunokawa, M., Wakahara, T., Onda, Y. (2014). Soil removal as a decontamination practice and radiocesium accumulation in tadpoles in rice paddies at Fukushima. Environmental Pollution, 187, 112–115. doi: https://doi.org/10.1016/j.envpol.2014.01.002
- Yasutaka, T., Naito, W. (2016). Assessing cost and effectiveness of radiation decontamination in Fukushima Prefecture, Japan. Journal of Environmental Radioactivity, 151, 512–520. doi: https://doi.org/10.1016/j.jenvrad.2015.05.012
- Nakayama, S., Kawase, K., Hardie, S., Yashio, S., Iijima, K., Mckinley, I. et al. (2015). Remediation of Contaminated Areas in the Aftermath of the Accident at the Fukushima Daiichi Nuclear Power Station: Overview, Analysis and Lessons Learned. Japan Atomic Energy Agency. JAEA-Review, 60.
- Comans, R. N. J. (1997). Kinetics and reversibility of radiocaesium solid/liquid partitioning in sediments. ECN report number: ECN-RX--97-044, 20.
- Liu, C., Zachara, J. M., Smith, S. C., McKinley, J. P., Ainsworth, C. C. (2003). Desorption kinetics of radiocesium from subsurface sediments at Hanford Site, USA. Geochimica et Cosmochimica Acta, 67 (16), 2893–2912. doi: https://doi.org/10.1016/s0016-7037(03)00267-9
- Kim, J.-H., Kim, S.-M., Yoon, I.-H., Yang, H.-M., Kim, I. (2021). Novel two-step process for remediation of Cs-contaminated soil assisted by magnetic composites. Chemical Engineering Journal, 424, 130554. doi: https://doi.org/10.1016/j.cej.2021.130554
- Nikolaevskii, V. B., Poluektov, P. P., Arustamov, A. E. (2011). Perspektivy razvitiia tekhnologii dezaktivatcii gruntov. Bezopasnost iadernykh tekhnologii i okruzhaiushchei sredy, 4, 114–117.
- Petrov, S. V., Zabulonov, Y. L., Homma, M. (2021). Study on Plasma-Stimulated Remediation of Radioactively Contaminated Soil. New Approaches in Engineering Research, 3, 103–115. doi: https://doi.org/10.9734/bpi/naer/v3/10209d
- Petrov, S. V. (2013). Plazmennaia ochistka vody i grunta ot tiazhelykh metallov i radionuklidov. Energotekhnologii i resursosberezhenie, 5, 38–46.
- Petrov, S. V., Katircioğlu, T. Y. (2020). Technological Aspects of Steam and Water Plasma. OmniSkriptum Publishing Group, 481.
- Koarashi, J., Nishimura, S., Atarashi-Andoh, M., Muto, K., Matsunaga, T. (2019). A new perspective on the 137Cs retention mechanism in surface soils during the early stage after the Fukushima nuclear accident. Scientific Reports, 9 (1). doi: https://doi.org/10.1038/s41598-019-43499-7
- Rezaei, F., Vanraes, P., Nikiforov, A., Morent, R., De Geyter, N. (2019). Applications of Plasma-Liquid Systems: A Review. Materials, 12 (17), 2751. doi: https://doi.org/10.3390/ma12172751
- Šimečková, J., Krčma, F., Klofáč, D., Dostál, L., Kozáková, Z. (2020). Influence of Plasma-Activated Water on Physical and Physical–Chemical Soil Properties. Water, 12 (9), 2357. doi: https://doi.org/10.3390/w12092357
- Barjasteh, A., Dehghani, Z., Lamichhane, P., Kaushik, N., Choi, E. H., Kaushik, N. K. (2021). Recent Progress in Applications of Non-Thermal Plasma for Water Purification, Bio-Sterilization, and Decontamination. Applied Sciences, 11 (8), 3372. doi: https://doi.org/10.3390/app11083372
- Foster, J. E. (2017). Plasma-based water purification: Challenges and prospects for the future. Physics of Plasmas, 24 (5), 055501. doi: https://doi.org/10.1063/1.4977921
- Kosenkov, V. M. (2011). Rezonansnye kolebaniia tcilindricheskoi stenki razriadnoi kamery v rezultate elektricheskogo razriada v vode. Prikladnaia mekhanika i tekhnicheskaia fizika, 52 (4), 43–51.
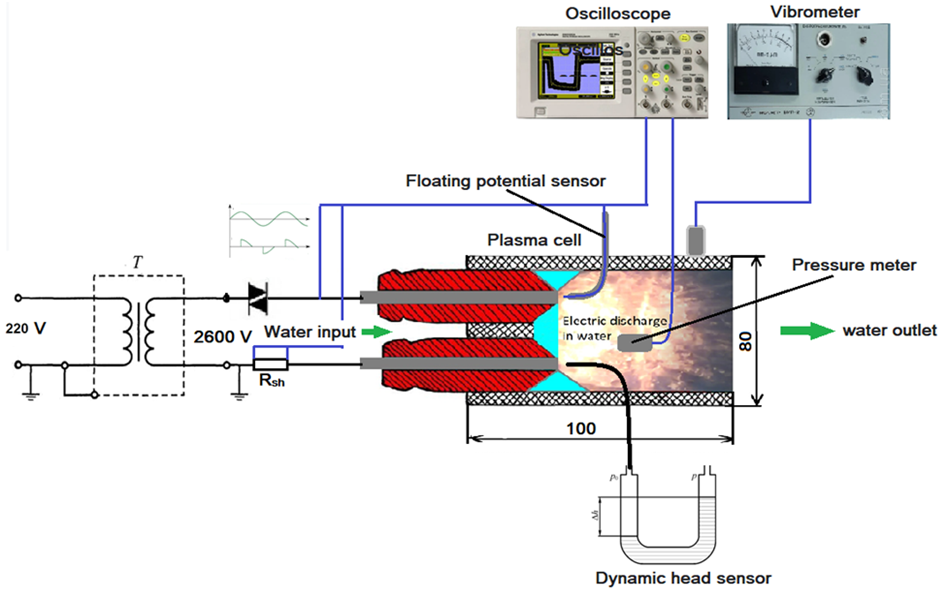
Downloads
Published
How to Cite
Issue
Section
License
Copyright (c) 2023 Stanislav Petrov, Serhii Bondarenko, Masato Homma

This work is licensed under a Creative Commons Attribution 4.0 International License.
The consolidation and conditions for the transfer of copyright (identification of authorship) is carried out in the License Agreement. In particular, the authors reserve the right to the authorship of their manuscript and transfer the first publication of this work to the journal under the terms of the Creative Commons CC BY license. At the same time, they have the right to conclude on their own additional agreements concerning the non-exclusive distribution of the work in the form in which it was published by this journal, but provided that the link to the first publication of the article in this journal is preserved.