Analysis of modern atmospheric electrostatic field measuring instruments and methods
DOI:
https://doi.org/10.15587/2706-5448.2023.285963Keywords:
electrostatic measurements, atmospheric electric field, electrostatic field, measuring instruments, electrostatic field millAbstract
The object of research is the process of measuring the strength of the atmospheric electrostatic field. This paper is devoted to an analytical review and comparative analysis of modern methods and instruments for measuring atmospheric electrostatic field strength. The results of scientific research and modern practical technologies, which are used to develop technical means and increase the accuracy of measuring the strength of electrostatic fields, are considered.
In the work, the general functional requirements for the hardware of systems for measuring the atmospheric electrostatic field strength are formed and the main directions of research and practical tasks for its creation are defined. The design features and characteristics of existing measuring instruments are considered in detail. The advantages and disadvantages of electrometers, electrostatic field mills, microelectromechanical electrostatic field mills, and electric field imaging systems are determined given their portability, sensitivity, measuring frequency, accuracy, measurement range, linearity, and cost. The analysis of the state of modern methods and measuring instruments for the strength of the electrostatic field showed that one of the best solutions for measuring the electrostatic field strength of the atmosphere today is the use of an improved electrostatic field mill.
It was determined that one of the important problems for ensuring the development of methods and means of atmospheric electrostatic field strength measuring is the need to generalize the structure of the measuring instruments and calculate its metrological characteristics. It has been established that solving the problem of increasing the accuracy of atmospheric electrostatic field strength measurement requires a comprehensive approach based on improving the design of the sensor structure of the meter, increasing the accuracy of navigation and positioning, increasing the autonomy of work, improving communication and data transmission systems, as well as ensuring high stability and reliability of work under the influence of external factors. Improving the structure and improving the characteristics of electrostatic field mills in the future will ensure the necessary accuracy, compactness, and availability for measurement and its inclusion in the automated system of atmospheric electrostatic field monitoring and forecasting.
References
- Whipple, F. J. W. (2007). Modern views on atmospheric electricity. Quarterly Journal of the Royal Meteorological Society, 64 (275), 199–222. doi: https://doi.org/10.1002/qj.49706427502
- Roble, R., Tzur, I. (1986). The global atmospheric electrical circuit. The Earth's electrical environment. Washington: National Academies Press, 206–231.
- Markson, R. (2007). The Global Circuit Intensity: Its Measurement and Variation over the Last 50 Years. Bulletin of the American Meteorological Society, 88 (2), 223–242. doi: https://doi.org/10.1175/bams-88-2-223
- Liu, C., Williams, E. R., Zipser, E. J., Burns, G. (2010). Diurnal Variations of Global Thunderstorms and Electrified Shower Clouds and Their Contribution to the Global Electrical Circuit. Journal of the Atmospheric Sciences, 67 (2), 309–323. doi: https://doi.org/10.1175/2009jas3248.1
- Blakeslee, R. J., Mach, D. M., Bateman, M. G., Bailey, J. C. (2014). Seasonal variations in the lightning diurnal cycle and implications for the global electric circuit. Atmospheric Research, 135-136, 228–243. doi: https://doi.org/10.1016/j.atmosres.2012.09.023
- Anisimov, S. V., Galichenko, S. V., Aphinogenov, K. V., Prokhorchuk, A. A. (2017). Evaluation of the Atmospheric Boundary-Layer Electrical Variability. Boundary-Layer Meteorology, 327–348. doi: https://doi.org/10.1007/s10546-017-0328-0
- Jacobson, E. A., Krider, E. P. (1976). Electrostatic Field Changes Produced by Florida Lightning. Journal of the Atmospheric Sciences, 33 (1), 103–117. doi: https://doi.org/10.1175/1520-0469(1976)033<0103:efcpbf>2.0.co;2
- Koshak, W. J., Krider, E. P. (1989). Analysis of lightning field changes during active Florida thunderstorms. Journal of Geophysical Research, 94 (D1), 1165. doi: https://doi.org/10.1029/jd094id01p01165
- Koshak, W. J., Krider, E. P. (1994). A Linear Method for Analyzing Lightning Field Changes. Journal of the Atmospheric Sciences, 51 (4), 473–488. doi: https://doi.org/10.1175/1520-0469(1994)051<0473:almfal>2.0.co;2
- Maier, L. M., Krider, E. P. (1986). The charges that are deposited by cloud-to-ground lightning in Florida. Journal of Geophysical Research, 91 (D12), 13275. doi: https://doi.org/10.1029/jd091id12p13275
- Murphy, M. J., Krider, E. P., Maier, M. W. (1996). Lightning charge analyses in small Convection and Precipitation Electrification (CaPE) experiment storms. Journal of Geophysical Research: Atmospheres, 101 (D23), 29615–29626. Portico. doi: https://doi.org/10.1029/96jd01538
- Chubb, J., Harbour, J. (2000). A system for the advance warning of risk of lightning. Paper presented at the Electrostatics Society of America ‘ESA 2000’ meeting Niagara Falls. Niagara Falls.
- Montanya, J., Bergas, J., Hermoso, B. (2004). Electric field measurements at ground level as a basis for lightning hazard warning. Journal of Electrostatics, 60 (2-4), 241–246. doi: https://doi.org/10.1016/j.elstat.2004.01.009
- Murphy, M. J., Holle, R. L., Demetriades, N. W. S. (2008). Cloud-to-ground lightning warnings using electric field mill and lightning observations. 20th International Lightning Detection Conference (ILDC). Tucson.
- 7 Electrostatic Instrument Manufacturers in 2023. Metoree. Available at: https://us.metoree.com/categories/static-electricity-meter/
- Electrostatic field measuring instrument max. ±30 kV | AD-1684A. DirectIndustry. Available at: https://www.directindustry.com/prod/d-company-limited/product-54946-1443153.html
- GHRC: Lightning field campaigns, detection instruments, and research proposals. Wayback Machine. Aviailable at: https://web.archive.org/web/20160307112621/http://thunder.msfc.nasa.gov/validation/validation.html#Interp
- Bateman, M. G., Stewart, M. F., Podgorny, S. J., Christian, H. J., Mach, D. M., Blakeslee, R. J. et al. (2007). A Low-Noise, Microprocessor-Controlled, Internally Digitizing Rotating-Vane Electric Field Mill for Airborne Platforms. Journal of Atmospheric and Oceanic Technology, 24 (7), 1245–1255. doi: https://doi.org/10.1175/jtech2039.1
- Chauzy, S., Médale, J.-C., Prieur, S., Soula, S. (1991). Multilevel measurement of the electric field underneath a thundercloud: 1. A new system and the associated data processing. Journal of Geophysical Research, 96 (D12), 22319. doi: https://doi.org/10.1029/91jd02031
- Winn, W. P., Moore, C. B. (1971). Electric field measurements in thunderclouds using instrumented rockets. Journal of Geophysical Research, 76 (21), 5003–5017. doi: https://doi.org/10.1029/jc076i021p05003
- Ackermann, L., Bouwers, A., Carlsson, C., Dümmlin, K., Goering, U., Haxel, O. et al. (1968). Encyclopedia of Medical Radiology. Verlag Berlin Heidelberg.
- Acharya, Y. B. (2000). A wide range linear electrometer. Review of Scientific Instruments, 71 (6), 2585–2588. doi: https://doi.org/10.1063/1.1150653
- Harrison, R. G. (1997). An antenna electrometer system for atmospheric electrical measurements. Review of Scientific Instruments, 68 (3), 1599–1603. doi: https://doi.org/10.1063/1.1147932
- Harrison, R. G., Marlton, G. J., Nicoll, K. A., Airey, M. W., Williams, P. D. (2017). A self-calibrating wide range electrometer for in-cloud measurements. Review of Scientific Instruments, 88 (12). doi: https://doi.org/10.1063/1.5011177
- Antunes de Sá, A., Marshall, R., Sousa, A., Viets, A., Deierling, W. (2020). An Array of Low‐Cost, High‐Speed, Autonomous Electric Field Mills for Thunderstorm Research. Earth and Space Science, 7 (11). doi: https://doi.org/10.1029/2020ea001309
- Cui, Y., Yuan, H., Song, X., Zhao, L., Liu, Y., Lin, L. (2018). Model, Design, and Testing of Field Mill Sensors for Measuring Electric Fields Under High-Voltage Direct-Current Power Lines. IEEE Transactions on Industrial Electronics, 65 (1), 608–615. doi: https://doi.org/10.1109/tie.2017.2719618
- Buguet, M., Lalande, P., Laroche, P., Blanchet, P., Bouchard, A., Chazottes, A. (2021). Thundercloud Electrostatic Field Measurements during the Inflight EXAEDRE Campaign and during Lightning Strike to the Aircraft. Atmosphere, 12 (12), 1645. doi: https://doi.org/10.3390/atmos12121645
- Hsu, C. H., Muller, R. S. (1991). Micromechanical electrostatic voltmeter. TRANSDUCERS ’91: 1991 International Conference on Solid-State Sensors and Actuators. Digest of Technical Papers. San Francisco. doi: https://doi.org/10.1109/sensor.1991.148966
- Yong Zhu, Lee, J. E.-Y., Seshia, A. A. (2008). A Resonant Micromachined Electrostatic Charge Sensor. IEEE Sensors Journal, 8 (9), 1499–1505. doi: https://doi.org/10.1109/jsen.2008.923597
- Peng, C., Chen, X., Bai, Q., Luo, L., Xia, S. (2006). A novel high performance micromechanical resonant electrostatic field sensor used in atmospheric electric field detection. Proceedings of the IEEE International Conference on MICRO Electro Mechanical Systems. Las Vegas. doi: https://doi.org/10.1109/memsys.2006.1627895
- Chen, X., Peng, C., Xia, S. (2008). Design of a thermally driven resonant miniature electric field sensor with feedback control. Proceedings of the IEEE International Conference on Nano/micro Engineered and Molecular Systems. Sanya. doi: https://doi.org/10.1109/nems.2008.4484329
- Riehl, P. S., Scott, K. L., Muller, R. S., Howe, R. T., Yasaitis, J. A. (2003). Electrostatic charge and field sensors based on micromechanical resonators. Journal of Microelectromechanical Systems, 12 (5), 577–589. doi: https://doi.org/10.1109/jmems.2003.818066
- Gong, C., Tao, H., Peng, C., Bai, Q., Chen, S., Xia, S. (2005). A novel miniature interlacing vibrating electric field sensor. Proceedings of the IEEE Sensors. Irvine. doi: https://doi.org/10.1109/icsens.2005.1597722
- Yang, P., Peng, C., Zhang, H., Liu, S., Fang, D., Xia, S. (2011). A high sensitivity SOI electric-field sensor with novel comb-shaped microelectrodes. Proceedings of the 16th International Solid-State Sensors, Actuators and Microsystems Conference. Beijing. doi: https://doi.org/10.1109/transducers.2011.5969165
- Horenstein, M. N., Stone, P. R. (2001). A micro-aperture electrostatic field mill based on MEMS technology. Journal of Electrostatics, 51-52, 515–521. doi: https://doi.org/10.1016/s0304-3886(01)00048-1
- Bahreyni, B., Wijeweera, G., Shafai, C., Rajapakse, A. (2007). Design and testing of a field-chopping electric field sensor using thermal actuators with mechanically amplified response. Proceedings of the Solid-State Sensors, Actuators and Microsystems Conference. Lyon. doi: https://doi.org/10.1109/sensor.2007.4300404
- Bahreyni, B., Wijeweera, G., Shafai, C., Rajapakse, A. (2008). Analysis and Design of a Micromachined Electric-Field Sensor. Journal of Microelectromechanical Systems, 17 (1), 31–36. doi: https://doi.org/10.1109/jmems.2007.911870
- Huang, J., Wu, X., Wang, X., Yan, X., Lin, L. (2015). A novel high-sensitivity electrostatic biased electric field sensor. Journal of Micromechanics and Microengineering, 25 (9), 095008. doi: https://doi.org/10.1088/0960-1317/25/9/095008
- Wang, Y., Fang, D., Feng, K., Ren, R., Chen, B., Peng, C., Xia, S. (2015). A novel micro electric field sensor with X–Y dual axis sensitive differential structure. Sensors and Actuators A: Physical, 229, 1–7. doi: https://doi.org/10.1016/j.sna.2015.03.013
- Ma, Q., Huang, K., Yu, Z., Wang, Z. (2017). An electric field sensor with double-layer floating structure for measurement of dc synthetic field coupled with ion flow. Proceedings of the International Conference on Solid-State Sensors, Actuators and Microsystems. Kaohsiung. doi: https://doi.org/10.1109/transducers.2017.7994192
- Underwood, G. C. (2019). A MEMS Dual Vertical Electrometer and Electric Field-Mill. Aviailable at: https://scholar.afit.edu/etd/2288
- Ling, B., Wang, Y., Peng, C., Li, B., Chu, Z., Li, B., Xia, S. (2017). Single-chip 3D electric field microsensor. Frontiers of Mechanical Engineering, 12 (4), 581–590. doi: https://doi.org/10.1007/s11465-017-0454-x
- Chu, Z., Peng, C., Ren, R., Ling, B., Zhang, Z., Lei, H., Xia, S. (2018). A High Sensitivity Electric Field Microsensor Based on Torsional Resonance. Sensors, 18 (1), 286. doi: https://doi.org/10.3390/s18010286
- Smith, J. R. (1999). Electric field imaging. Massachusetts Institute of Technology.
- Smith, J. R., Garcia, E., Wistort, R., Krishnamoorthy, G. (2007). Electric field imaging pretouch for robotic graspers. 2007 IEEE/RSJ International Conference on Intelligent Robots and Systems, 676–683. doi: https://doi.org/10.1109/iros.2007.4399609
- Electric Field Imaging System. T2 Portal. NASA Technology Transfer Portal Home. Available at: https://technology.nasa.gov/patent/LAR-TOPS-116 last accessed: 15.07.2023
- Generazio, E. R. (2017). Electric potential and electric field imaging. AIP Conference Proceedings. AIP Publishing, 1806 (1). doi: https://doi.org/10.1063/1.4974566
- Generazio, E. R. (2016). Pat. No. 9,279,719 USA. Electric field quantitative measurement system and method. 08.05.2016.
- Generazio, E. R. (2017). Pat. No. 9,804,199 USA. Ephemeral electric potential and electric field sensor. 31.10.2017.
- Generazio, E. R. (2017). Pat. No. 9,559,616 USA. Quasi-static electric field generator. 31.01.2017.
- Generazio, E. R. (2020). Pat. No. 10,712,378 USA. Dynamic multidimensional electric potential and electric field quantitative measurement system and method. 14.07.2020.
- Generazio, E. R. (2021). Pat. No. 10,900,930 USA. Method for phonon assisted creation and annihilation of subsurface electric dipoles. 26.01.2021.
- Generazio, E. R. (2022). Pat. No. 11,293,964 USA. Dynamic multidimensional electric potential and electric field quantitative measurement system and method. 05.04.2022.
- Generazio, E. R. (2022). Pat. No. 11,360,048 USA. Method for phonon assisted creation and annihilation of subsurface electric dipoles. 14.07.2022.
- Mens, L. H. M. (2007). Advances in Cochlear Implant Telemetry: Evoked Neural Responses, Electrical Field Imaging, and Technical Integrity. Trends in Amplification, 11 (3), 143–159. doi: https://doi.org/10.1177/1084713807304362
- NASA Technical Reports Server (NTRS). Available at: https://ntrs.nasa.gov/api/citations/20160008937/downloads/20160008937.pdf
- Generazio, E. R. (2019). Pat. No. 10,281,430 USA. Identification and characterization of remote objects by electric charge tunneling, injection, and induction, and an erasable organic molecular memory. 07.05.2019.
- Generazio, E. R. (2020). Pat. No. 10,620,252 USA. Electric field imaging system. 14.04.2020.
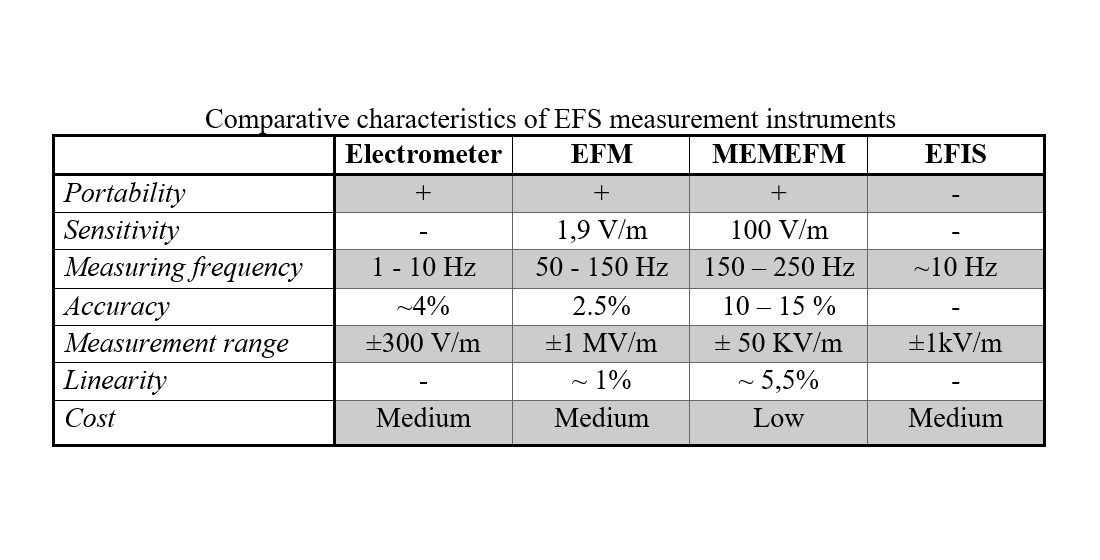
Downloads
Published
How to Cite
Issue
Section
License
Copyright (c) 2023 Oleksandr Povcshenko, Viktor Bazhenov

This work is licensed under a Creative Commons Attribution 4.0 International License.
The consolidation and conditions for the transfer of copyright (identification of authorship) is carried out in the License Agreement. In particular, the authors reserve the right to the authorship of their manuscript and transfer the first publication of this work to the journal under the terms of the Creative Commons CC BY license. At the same time, they have the right to conclude on their own additional agreements concerning the non-exclusive distribution of the work in the form in which it was published by this journal, but provided that the link to the first publication of the article in this journal is preserved.