Analysis of the structure of electrically conductive composite ceramics
DOI:
https://doi.org/10.15587/2706-5448.2024.297612Keywords:
electrically conductive ceramics, microstructure, silicon carbide, SiC, porosity, electrical insulation properties, electron microscopyAbstract
The object of the research is electrically conductive ceramics. It aims to analyze the microstructure of electrically conductive ceramic composites based on silicon carbide and investigate the influence of silicon carbide content on their properties. This study is pivotal for enhancing materials used in high-tech applications, particularly in fields where distinct electrical insulation and mechanical characteristics are crucial. The microstructure analysis conducted through scanning electron microscopy confirmed the presence of silicon carbide in all examined ceramic samples, except in those where silicon carbide was not added. Special attention should be given to the sample with 30 % silicon carbide, distinguished by the lowest open porosity. These findings are corroborated by previous research where this sample exhibited superior properties: open porosity – 12.51 %, water absorption – 5.88 %, apparent density – 2.13 g/cm³, specific resistance – 0.43·106 Ω·m. These characteristics indicate low porosity and high structural-physical property values. The results not only affirm the successful incorporation of silicon carbide into the ceramic matrix but also highlight the prospects for applying the researched ceramic materials in areas where electrical insulation and mechanical properties are crucial. Specifically, the sample with 30 % silicon carbide appears particularly promising due to its high characteristics and lower porosity, making it potentially interesting for applications in high-tech industries such as electronics and telecommunications. The conclusions suggest the potential use of these ceramic materials in various high-tech industries where both electrical and mechanical properties are essential. The sample with 30 % silicon carbide, with its exceptional characteristics, holds potential for applications in advanced technologies. Further research in this direction could lead to the development of new materials for effective radiofrequency absorption, finding broad applications in different technological fields.
References
- Tang, W., Lu, L., Xing, D., Fang, H., Liu, Q., Teh, K. S. (2018). A carbon-fabric/polycarbonate sandwiched film with high tensile and EMI shielding comprehensive properties: An experimental study. Composites Part B: Engineering, 152, 8–16. doi: https://doi.org/10.1016/j.compositesb.2018.06.026
- Khodiri, A. A., Al-Ashry, M. Y., El-Shamy, A. G. (2020). Novel hybrid nanocomposites based on polyvinyl alcohol/graphene/magnetite nanoparticles for high electromagnetic shielding performance. Journal of Alloys and Compounds, 847. doi: https://doi.org/10.1016/j.jallcom.2020.156430
- Yim, Y.-J., Rhee, K. Y., Park, S.-J. (2016). Electromagnetic interference shielding effectiveness of nickel-plated MWCNTs/high-density polyethylene composites. Composites Part B: Engineering, 98, 120–125. doi: https://doi.org/10.1016/j.compositesb.2016.04.061
- Sun, X., Liu, X., Shen, X., Wu, Y., Wang, Z., Kim, J.-K. (2016). Graphene foam/carbon nanotube/poly(dimethyl siloxane) composites for exceptional microwave shielding. Composites Part A: Applied Science and Manufacturing, 85, 199–206. doi: https://doi.org/10.1016/j.compositesa.2016.03.009
- Chung, D. D. L. (2000). Materials for Electromagnetic Interference Shielding. Journal of Materials Engineering and Performance, 9 (3), 350–354. doi: https://doi.org/10.1361/105994900770346042
- Xia, F., Xia, Y., Chen, S., Chen, L., Zhu, W., Chen, Y. et al. (2017). Erratum to: Lipid emulsion mitigates impaired pulmonary function induced by limb I/R in rats through attenuation of local cellular injury and the subsequent systemic inflammatory response/inflammation. BMC Anesthesiology, 17 (1). doi: https://doi.org/10.1186/s12871-017-0407-2
- Gargama, H., Thakur, A. K., Chaturvedi, S. K. (2016). Polyvinylidene fluoride/nanocrystalline iron composite materials for EMI shielding and absorption applications. Journal of Alloys and Compounds, 654, 209–215. doi: https://doi.org/10.1016/j.jallcom.2015.09.059
- Wang, L., Chen, L., Song, P., Liang, C., Lu, Y., Qiu, H. et al. (2019). Fabrication on the annealed Ti3C2Tx MXene/Epoxy nanocomposites for electromagnetic interference shielding application. Composites Part B: Engineering, 171, 111–118. doi: https://doi.org/10.1016/j.compositesb.2019.04.050
- Geetha, S., Satheesh Kumar, K. K., Rao, C. R. K., Vijayan, M., Trivedi, D. C. (2009). EMI shielding: Methods and materials – A review. Journal of Applied Polymer Science, 112 (4), 2073–2086. doi: https://doi.org/10.1002/app.29812
- Saini, P., Arora, M., Gupta, G., Gupta, B. K., Singh, V. N., Choudhary, V. (2013). High permittivity polyaniline–barium titanate nanocomposites with excellent electromagnetic interference shielding response. Nanoscale, 5 (10), 4330. doi: https://doi.org/10.1039/c3nr00634d
- Thomassin, J.-M., Jérôme, C., Pardoen, T., Bailly, C., Huynen, I., Detrembleur, C. (2013). Polymer/carbon based composites as electromagnetic interference (EMI) shielding materials. Materials Science and Engineering: R: Reports, 74 (7), 211–232. doi: https://doi.org/10.1016/j.mser.2013.06.001
- Joseph, A. M. (2013). The stage 3 meaningful use preliminary recommendations: concerns are being raised. MLO Med. Lab. Obs., 45 (7), 64.
- Duan, S., Zhu, D., Zhou, W., Luo, F., Chen, Q. (2020). Mechanical and microwave absorption properties of SiCf/SiC–Al4C3 composite with EPD-SiO2/ZrO2 interphase prepared by precursor infiltration and active filler-controlled pyrolysis method. Ceramics International, 46 (8), 12344–12352. doi: https://doi.org/10.1016/j.ceramint.2020.01.285
- Katoh, Y., Snead, L. L., Henager, C. H., Nozawa, T., Hinoki, T., Iveković, A., Novak, S., Gonzalez de Vicente, S. M. (2014). Current status and recent research achievements in SiC/SiC composites. Journal of Nuclear Materials, 455 (1-3), 387–397. doi: https://doi.org/10.1016/j.jnucmat.2014.06.003
- Morscher, G. N. (2004). Stress-dependent matrix cracking in 2D woven SiC-fiber reinforced melt-infiltrated SiC matrix composites. Composites Science and Technology, 64 (9), 1311–1319. doi: https://doi.org/10.1016/j.compscitech.2003.10.022
- Lisachuk, G. V., Sakhnenko, N. D., Pitak, Ya. M., Krivobok, R. V., Maystat, M. S., Zakharov, A. V. et al. (2021). Creation of electrically conductive composite ceramics based on facing tiles with the addition of SiC. Scientific Research on Refractories and Technical Ceramics, 121, 121–128. doi: https://doi.org/10.35857/2663-3566.121.13
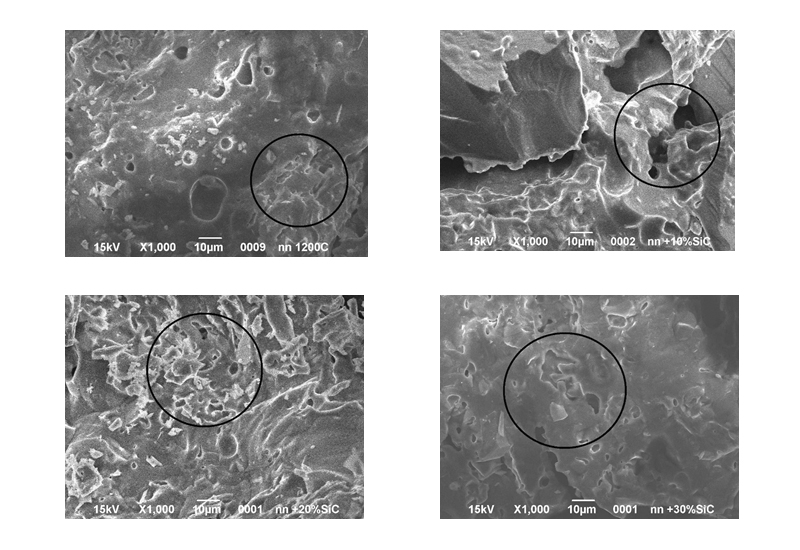
Downloads
Published
How to Cite
Issue
Section
License
Copyright (c) 2024 Mykyta Maistat, Andrii Kryvobok

This work is licensed under a Creative Commons Attribution 4.0 International License.
The consolidation and conditions for the transfer of copyright (identification of authorship) is carried out in the License Agreement. In particular, the authors reserve the right to the authorship of their manuscript and transfer the first publication of this work to the journal under the terms of the Creative Commons CC BY license. At the same time, they have the right to conclude on their own additional agreements concerning the non-exclusive distribution of the work in the form in which it was published by this journal, but provided that the link to the first publication of the article in this journal is preserved.