Adaptive hybrid numerical modeling of wave processes in multilayer structures based on TMM and FEM methods
DOI:
https://doi.org/10.15587/2706-5448.2025.323919Keywords:
numerical modeling, multilayered structures, wave processes, adaptive algorithms, hybrid approach, grid discretizationAbstract
The object of research in this work is wave processes in multilayer thin films and methods of their numerical modeling using adaptive hybrid models. The research covers multilayer media with gradient distribution of physical parameters, including inhomogeneities.
The problem addressed in this study is the enhancement of the accuracy and efficiency of numerical modeling of wave processes in complex multilayered structures while reducing computational costs. Traditional methods, such as the transfer matrix method or the finite element method, have limitations related to computational complexity, numerical stability, and the ability to account for intricate geometric features.
The essence of the obtained results lies in the development and software implementation of an adaptive hybrid model that combines the transfer matrix method for wave propagation calculations in homogeneous regions and the finite element method for modeling complex geometries. The proposed approach optimizes computational resources by dynamically adjusting the grid resolution according to local variations in the refractive index. The use of adaptive discretization reduced the number of computational points by 40 % without compromising calculation accuracy. The relative error of the results obtained using the proposed model does not exceed 1 %, demonstrating its high precision.
The achieved results can be attributed to the implementation of efficient adaptive algorithms that automatically adjust the grid resolution based on the gradient of physical parameters, as well as the application of consistent boundary conditions between computational domains using different methods. This ensures a smooth transition between different modeling zones and minimizes numerical errors at domain boundaries.
The practical applications of these findings include optical technologies for the design and optimization of photonic devices, sensors, anti-reflective coatings, and nanostructured materials. The model can be utilized for the analysis of complex multilayered systems in nanotechnology, biomedical research, and the design of micro-optical elements. It is particularly useful in scenarios where it is necessary to account for structural inhomogeneities, complex geometries, and boundary conditions while maintaining minimal computational costs.
References
- Dhaouadi, A., Bousselmi, K., Monnet, S., Gammoudi, M. M., Hammoudi, S. (2022). A Multi-layer Modeling for the Generation of New Architectures for Big Data Warehousing. Advanced Information Networking and Applications, 204–218. https://doi.org/10.1007/978-3-030-99587-4_18
- Lebedev, O. V., Ozerin, A. N., Abaimov, S. G. (2021). Multiscale Numerical Modeling for Prediction of Piezoresistive Effect for Polymer Composites with a Highly Segregated Structure. Nanomaterials, 11 (1), 162. https://doi.org/10.3390/nano11010162
- Clingerman, M. L., King, J. A., Schulz, K. H., Meyers, J. D. (2001). Evaluation of electrical conductivity models for conductive polymer composites. Journal of Applied Polymer Science, 83 (6), 1341–1356. https://doi.org/10.1002/app.10014
- Agarwal, M., Pasupathy, P., Wu, X., Recchia, S. S., Pelegri, A. A. (2024). Multiscale Computational and Artificial Intelligence Models of Linear and Nonlinear Composites: A Review. Small Science, 4 (5). https://doi.org/10.1002/smsc.202300185
- Zhu, X. Y., Lee, J. H., Kim, K.-H., Lim, C.-H., Lee, S. H. (2024). Coupled CFD modeling and thermal analysis of multi-layered insulation structures in liquid hydrogen storage tanks for various vapor-cooled shields. Case Studies in Thermal Engineering, 63, 105317. https://doi.org/10.1016/j.csite.2024.105317
- Šeta, B., Sandberg, M., Brander, M., Mollah, Md. T., Pokkalla, D. K., Kumar, V., Spangenberg, J. (2024). Numerical modeling of fiber orientation in multi-layer, isothermal material-extrusion big area additive manufacturing. Additive Manufacturing, 92, 104396. https://doi.org/10.1016/j.addma.2024.104396
- Jaśkowiec, J., Stankiewicz, A., Pluciński, P. (2020). Three-dimensional numerical modelling of multi-layered shell structures using two-dimensional plane mesh. Advances in Engineering Software, 149, 102840. https://doi.org/10.1016/j.advengsoft.2020.102840
- Kasper, Y., Tuchin, A., Bokova, A., Bityutskaya, L. (2016). Numerical simulation of multi-layer graphene structures based on quantum-chemical model. Journal of Physics: Conference Series, 741, 012022. https://doi.org/10.1088/1742-6596/741/1/012022
- Yin, D., Xu, Z., Feng, J., Qin, Y. (2014). Numerical Modelling of Multilayered Coatings – Latest Developments and Applications. Manufacturing Review, 1, 8. https://doi.org/10.1051/mfreview/2014008
- Ying, P., Tian, X., Xia, Y. (2025). Plasticity analysis and a homogenized constitutive model of compressible multi-layer structure of battery. Composite Structures, 351, 118586. https://doi.org/10.1016/j.compstruct.2024.118586
- Zhao, L., Zhang, T., Gu, J., Wang, T., Xie, B., Gao, F. (2024). N-level complex helical structure modeling method. Scientific Reports, 14 (1). https://doi.org/10.1038/s41598-024-69246-1
- Chen, Jiayu., Yao, B., Lu, Q., Wang, X., Yu, P., Ge, H. (2024). A safety dynamic evaluation method for missile mission based on multi-layered safety control structure model. Reliability Engineering & System Safety, 241, 109678. https://doi.org/10.1016/j.ress.2023.109678
- Ferreira, M., Carvalho, V., Ribeiro, J., Lima, R. A., Teixeira, S., Pinho, D. (2024). Advances in Microfluidic Systems and Numerical Modeling in Biomedical Applications: A Review. Micromachines, 15 (7), 873. https://doi.org/10.3390/mi15070873
- Berger, S., Denner, M.-S., Roeglinger, M. (2018). The nature of digital technologies – Development of a multi-layer taxonomy. Research Papers, 92. Available at: https://aisel.aisnet.org/ecis2018_rp/92
- Repins, I., Contreras, M. A., Egaas, B., DeHart, C., Scharf, J., Perkins, C. L., To, B., Noufi, R. (2008). 19·9%‐efficient ZnO/CdS/CuInGaSe2 solar cell with 81·2% fill factor. Progress in Photovoltaics: Research and Applications, 16 (3), 235–239. Portico. https://doi.org/10.1002/pip.822
- Yeh, P. (1988). Optical Waves in Layered Media. Wiley.
- Yeh, P., Hendry, M. (1990). Optical Waves in Layered Media. Physics Today, 43 (1), 77–78. https://doi.org/10.1063/1.2810419
- Kotlyar, V. V. (2012). Planar Gradient Hyperbolic Secant Lens for Subwavelength Focusing and Superresolution Imaging. Optics, 1 (1). https://doi.org/10.11648/j.optics.20120101.11
- Taflove, A., Hagness, S. (2000). Computational electrodynamics: The finite-difference time-domain method. Artech House, Inc. Available at: https://www.researchgate.net/publication/202924434
- Shuaibov, O. K., Hrytsak, R. V., Minya, O. I., Malinina, A. A., Bilak, Yu. Yu., Gomoki, Z. T. (2022). Spectroscopic diagnostics of overstressed nanosecond discharge plasma between zinc electrodes in air and nitrogen. Journal of Physical Studies, 26 (2). https://doi.org/10.30970/jps.26.2501
- Shuaibov, A. K., Minya, A. I., Malinina, A. A., Gritsak, R. V., Malinin, A. N., Bilak, Yu. Yu., Vatrala, M. I. (2022). Characteristics and Plasma Parameters of the Overstressed Nanosecond Discharge in Air between an Aluminum Electrode and a Chalcopyrite Electrode (СuInSe2). Surface Engineering and Applied Electrochemistry, 58 (4), 369–385. https://doi.org/10.3103/s1068375522040123
- Bondar, I. I., Suran, V. V., Minya, O. Y., Shuaibov, O. K., Bilak, Yu. Yu., Shevera, I. V. et al. (2023). Synthesis of Surface Structures during Laser-Stimulated Evaporation of a Copper Sulfate Solution in Distilled Water. Ukrainian Journal of Physics, 68 (2), 138. https://doi.org/10.15407/ujpe68.2.138
- Shuaibov, O. K., Minya, O. Y., Hrytsak, R. V., Bilak, Yu. Yu., Malinina, A. O., Homoki, Z. T. et al. (2023). Gas Discharge Source of Synchronous Flows of UV Radiation and Silver Sulphide Microstructures. Physics and Chemistry of Solid State, 24 (3), 417–421. https://doi.org/10.15330/pcss.24.3.417-421
- Hrytsak, R., Shuaibov, O., Minya, O., Malinina, A., Shevera, I., Bilak, Y., Homoki, Z. (2024). Conditions for pulsed gas-discharge synthesis of thin tungsten oxide films from a plasma mixture of air with tungsten vapors. Physics and Chemistry of Solid State, 25 (4), 684–688. https://doi.org/10.15330/pcss.25.4.684-688
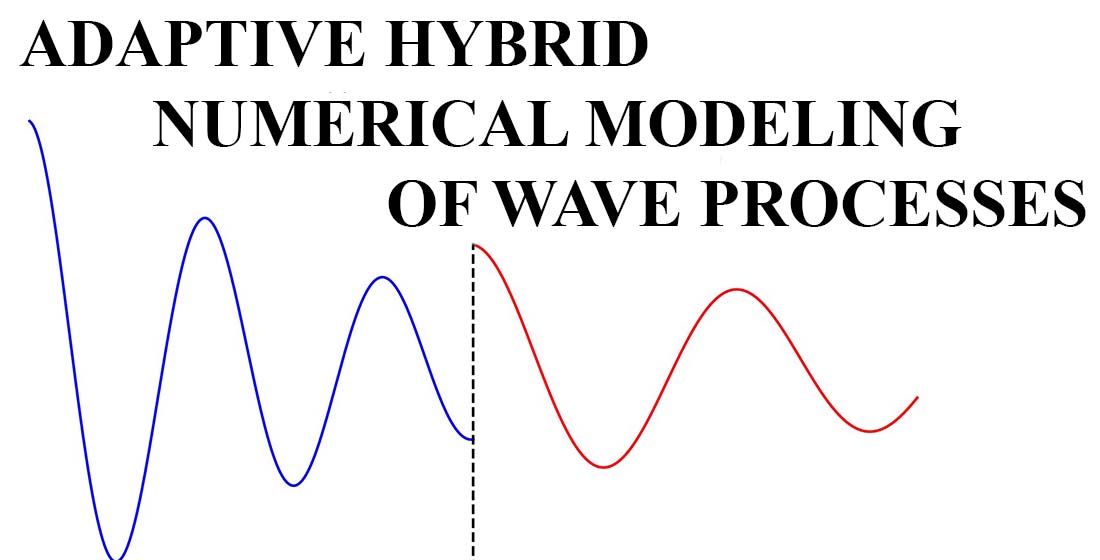
Downloads
Published
How to Cite
Issue
Section
License
Copyright (c) 2025 Yurii Bilak, Fedir Saibert, Roman Buchuk, Mariana Rol

This work is licensed under a Creative Commons Attribution 4.0 International License.
The consolidation and conditions for the transfer of copyright (identification of authorship) is carried out in the License Agreement. In particular, the authors reserve the right to the authorship of their manuscript and transfer the first publication of this work to the journal under the terms of the Creative Commons CC BY license. At the same time, they have the right to conclude on their own additional agreements concerning the non-exclusive distribution of the work in the form in which it was published by this journal, but provided that the link to the first publication of the article in this journal is preserved.