Оглядове дослідження сонячної башти із використанням різних теплоносіїв
DOI:
https://doi.org/10.15587/2706-5448.2022.267560Ключові слова:
сонячна енергія, концентровані сонячні установки, баштова сонячна електростанція, теплоносійАнотація
Об’єктом дослідження є розрізнення різних теплоносіїв (HTF) у концентрації сонячної енергії (CSP). Технології CSP отримують все більше уваги в ці роки через те, що світ стикається зі значними проблемами, особливо щодо екологічних проблем і зростаючого попиту на електроенергію. Країни світу наразі зобов’язуються пом’якшити кліматичні зміни та обмежити викиди парникових газів, щоб утримувати глобальну температуру нижче 2 °C. У результаті для виробництва електроенергії потрібні відновлювані джерела енергії. Однією з найбільш широко використовуваних технологій є сонячна башта, де дзеркала відбивають сонячне випромінювання в центральний приймач на вершині башти, який містить робочу рідину, відому як рідина для передачі тепла. HTF є одним із найважливіших компонентів баштових сонячних електростанцій, які використовуються для передачі та накопичення теплової енергії для виробництва електроенергії. Це дослідження зосереджено на HTF, який використовується в баштових сонячних електростанціях, і як він може вплинути на ефективність електростанції. HTF, які обговорюються в цьому дослідженні, це повітря, вода/пара, розплавлені солі, рідкий натрій та надкритичний CO2. Серед огляду HTF у системі сонячних башт результати дослідження показують, що повітря може досягати найвищої температури, тоді як рідкий натрій досягає найвищої загальної ефективності установки.
Спонсор дослідження
- Presentation of research in the form of publication through financial support in the form of a grant from SUES (Support to Ukrainian Editorial Staff).
Посилання
- Gomaa, M. R., Matarneh, G. A., Shalby, M., AL-Rawashdeh, H. A. (2021). A State of the art Review on a Thermochemical Conversion of Carbonaceous Materials: Production of Synthesis Gas by Co-Gasification Process-Part I. Current Alternative Energy, 4 (1), 26–46. doi: https://doi.org/10.2174/2405463104999200904115100
- Gomaa, M. R., Al-Dmour, N., AL-Rawashdeh, H. A., Shalby, M. (2020). Theoretical model of a fluidized bed solar reactor design with the aid of MCRT method and synthesis gas production. Renewable Energy, 148, 91–102. doi: https://doi.org/10.1016/j.renene.2019.12.010
- Gomaa, M. R., Mustafa, R. J., Rezk, H. (2019). An experimental implementation and testing of a concentrated hybrid photovoltaic/thermal system with monocrystalline solar cells using linear Fresnel reflected mirrors. International Journal of Energy Research, 43, 8660–8673. doi: https://doi.org/10.1002/er.4862
- Mubeen, I., Khan, M. S., Abid, M., Ratlamwala, T. A. H., Yan, M. (2021). Performance assessment of a solar tower assisted combined cycle power plant using supercritical carbon dioxide as a heat transfer fluid. International Journal of Exergy, 36 (1), 30. doi: https://doi.org/10.1504/ijex.2021.10040963
- Samir Benammar. (2020). A Review Study on the Modeling and Simulation of Solar Tower Power Plants. Journal of Solar Energy Research Updates, 7, 100–121. doi: https://doi.org/10.31875/2410-2199.2020.07.9
- AlJuhani, M., Gomaa, M. R., Mandourah, T. S., Oreijah, M. M. A. (2021). The Environmental Effects on the Photovoltaic Panel Power: Jeddah Case Study. Journal of Mechanical Engineering Research and Developments, 44 (6), 251–262. Available at: https://jmerd.net/06-2021-251-262/
- Al-Rawashdeh, H. A., Al-Hwaiti, M., Yaseen, A., Behiri, M. R. G. (2021). Influence of Partial Replacement of Cement by Various Percentage of Scoria in Self-Compacting Concrete on Thermal Conductivity in the Jordan Building Construction for Energy Saving. International Review of Mechanical Engineering (IREME), 15 (7), 385–393. doi: https://doi.org/10.15866/ireme.v15i7.20929
- Shalby, M., Elhanafi, A., Walker, P., Dorrell, D. G., Salah, A., Gomaa, M. R. (2021). Experimental Investigation of the Small-scale Fixed Multi-chamber OWC Device. Chinese Journal of Mechanical Engineering, 34 (1). doi: https://doi.org/10.1186/s10033-021-00641-9
- Concentrated solar power had a global total installed capacity of 6,451 MW in 2019 (2019). Available at: https://helioscsp.com/concentrated-solar-power-had-a-global-total-installed-capacity-of-6451-mw-in-2019/
- Adiyaman, G., Çolak, L., Horuz, İ. (2019). The Impact of Heat Transfer Fluids on the Sustainable Solutions for Solar Power Tower. 4th International Sustainable Buildings Symposium, 647–660. doi: https://doi.org/10.5772/intechopen.87836
- Zheng, M., Zapata, J., Asselineau, C.-A., Coventry, J., Pye, J. (2020). Analysis of tubular receivers for concentrating solar tower systems with a range of working fluids, in exergy-optimised flow-path configurations. Solar Energy, 211, 999–1016. doi: https://doi.org/10.1016/j.solener.2020.09.037
- Shagdar, E., Lougou, B. G., Shuai, Y., Anees, J., Damdinsuren, C., Tan, H. (2020). Performance analysis and techno-economic evaluation of 300 MW solar-assisted power generation system in the whole operation conditions. Applied Energy, 264, 114744. doi: https://doi.org/10.1016/j.apenergy.2020.114744
- Merchán, R. P., Santos, M. J., Medina, A., Calvo Hernández, A. (2022). High temperature central tower plants for concentrated solar power: 2021 overview. Renewable and Sustainable Energy Reviews, 155, 111828. doi: https://doi.org/10.1016/j.rser.2021.111828
- Yang, H., Li, J., Huang, Y., Kwan, T. H., Cao, J., Pei, G. (2020). Feasibility research on a hybrid solar tower system using steam and molten salt as heat transfer fluid. Energy, 205, 118094. doi: https://doi.org/10.1016/j.energy.2020.118094
- Adebayo, V. O., Olalekan, O. (2017). Solar thermal with Solar Tower. Available at: https://www.researchgate.net/publication/319471818_Solar_thermal_with_Solar_Tower_Power_generation
- Okonkwo, E. C., Okwose, C. F., Abid, M., Ratlamwala, T. A. H. (2018). Second-Law Analysis and Exergoeconomics Optimization of a Solar Tower–Driven Combined-Cycle Power Plant Using Supercritical CO2. Journal of Energy Engineering, 144 (3). doi: https://doi.org/10.1061/(asce)ey.1943-7897.0000534
- Mohammadi, K., McGowan, J. G., Saghafifar, M. (2019). Thermoeconomic analysis of multi-stage recuperative Brayton power cycles: Part I-hybridization with a solar power tower system. Energy Conversion and Management, 185, 898–919. doi: https://doi.org/10.1016/j.enconman.2019.02.012
- Agyekum, E. B., Adebayo, T. S., Bekun, F. V., Kumar, N. M., Panjwani, M. K. (2021). Effect of Two Different Heat Transfer Fluids on the Performance of Solar Tower CSP by Comparing Recompression Supercritical CO2 and Rankine Power Cycles, China. Energies, 14 (12), 3426. doi: https://doi.org/10.3390/en14123426
- Czaplicka, N., Grzegórska, A., Wajs, J., Sobczak, J., Rogala, A. (2021). Promising Nanoparticle-Based Heat Transfer Fluids – Environmental and Techno-Economic Analysis Compared to Conventional Fluids. International Journal of Molecular Sciences, 22 (17), 9201. doi: https://doi.org/10.3390/ijms22179201
- Li, Q., Bai, F., Yang, B., Wang, Y., Xu, L., Chang, Z. et. al. (2018). Dynamic simulations of a honeycomb ceramic thermal energy storage in a solar thermal power plant using air as the heat transfer fluid. Applied Thermal Engineering, 129, 636–645. doi: https://doi.org/10.1016/j.applthermaleng.2017.10.063
- Hassani, S. E., Ouali, H. A. L., Raillani, B., Moussaoui, M. A., Mezrhab, A., Amraqui, S. (2020). Thermal Performance of Solar Tower Using Air as Heat Transfer Fluid under MENA Region Climate. 2020 5th International Conference on Renewable Energies for Developing Countries (REDEC). doi: https://doi.org/10.1109/redec49234.2020.9163893
- Gasa, G., Lopez-Roman, A., Prieto, C., Cabeza, L. F. (2021). Life Cycle Assessment (LCA) of a Concentrating Solar Power (CSP) Plant in Tower Configuration with and without Thermal Energy Storage (TES). Sustainability, 13 (7), 3672. doi: https://doi.org/10.3390/su13073672
- Shatnawi, H., Lim, C. W., Ismail, F. B., Aldossary, A. (2021). An optimisation study of a solar tower receiver: the influence of geometry and material, heat flux, and heat transfer fluid on thermal and mechanical performance. Heliyon, 7 (7), e07489. doi: https://doi.org/10.1016/j.heliyon.2021.e07489
- Dincer, I., Rosen, M. A., Khalid, F. (2018). 3.16 Thermal Energy Production. Comprehensive Energy Systems, 3, 673–706. doi: https://doi.org/10.1016/b978-0-12-809597-3.00335-7
- Turchi, C. S., Vidal, J., Bauer, M. (2018). Molten salt power towers operating at 600–650 °C: Salt selection and cost benefits. Solar Energy, 164, 38–46. doi: https://doi.org/10.1016/j.solener.2018.01.063
- Rouibah, A., Benazzouz, D., Kouider, R., Al-Kassir, A., García-Sanz-Calcedo, J., Maghzili, K. (2018). Solar Tower Power Plants of Molten Salt External Receivers in Algeria: Analysis of Direct Normal Irradiation on Performance. Applied Sciences, 8 (8), 1221. doi: https://doi.org/10.3390/app8081221
- Yu, Q., Fu, P., Yang, Y., Qiao, J., Wang, Z., Zhang, Q. (2020). Modeling and parametric study of molten salt receiver of concentrating solar power tower plant. Energy, 200, 117505. doi: https://doi.org/10.1016/j.energy.2020.117505
- Sorgulu, F., Dincer, I. (2018). Design and analysis of a solar tower power plant integrated with thermal energy storage system for cogeneration. International Journal of Energy Research, 43 (12), 6151–6160. doi: https://doi.org/10.1002/er.4233
- Manzolini, G., Lucca, G., Binotti, M., Lozza, G. (2021). A two-step procedure for the selection of innovative high temperature heat transfer fluids in solar tower power plants. Renewable Energy, 177, 807–822. doi: https://doi.org/10.1016/j.renene.2021.05.153
- Polimeni, S., Binotti, M., Moretti, L., Manzolini, G. (2018). Comparison of sodium and KCl-MgCl2 as heat transfer fluids in CSP solar tower with sCO2 power cycles. Solar Energy, 162, 510–524. doi: https://doi.org/10.1016/j.solener.2018.01.046
- Liu, J., He, Y., Lei, X. (2019). Heat-Transfer Characteristics of Liquid Sodium in a Solar Receiver Tube with a Nonuniform Heat Flux. Energies, 12 (8), 1432. https://doi.org/10.3390/en12081432
- Aguilar, R., Valenzuela, L., Avila-Marin, A. L., Garcia-Ybarra, P. L. (2019). Simplified heat transfer model for parabolic trough solar collectors using supercritical CO2. Energy Conversion and Management, 196, 807–820. doi: https://doi.org/10.1016/j.enconman.2019.06.029
- Silva-Pérez, M. A. (2017). Solar power towers using supercritical CO2 and supercritical steam cycles, and decoupled combined cycles. Advances in Concentrating Solar Thermal Research and Technology, 383–402. doi: https://doi.org/10.1016/b978-0-08-100516-3.00017-4
- Saghafifar, M., Mohammadi, K., Powell, K. (2020). Design and analysis of a dual-receiver direct steam generator solar power tower plant with a flexible heliostat field. Sustainable Energy Technologies and Assessments, 39, 100698. doi: https://doi.org/10.1016/j.seta.2020.100698
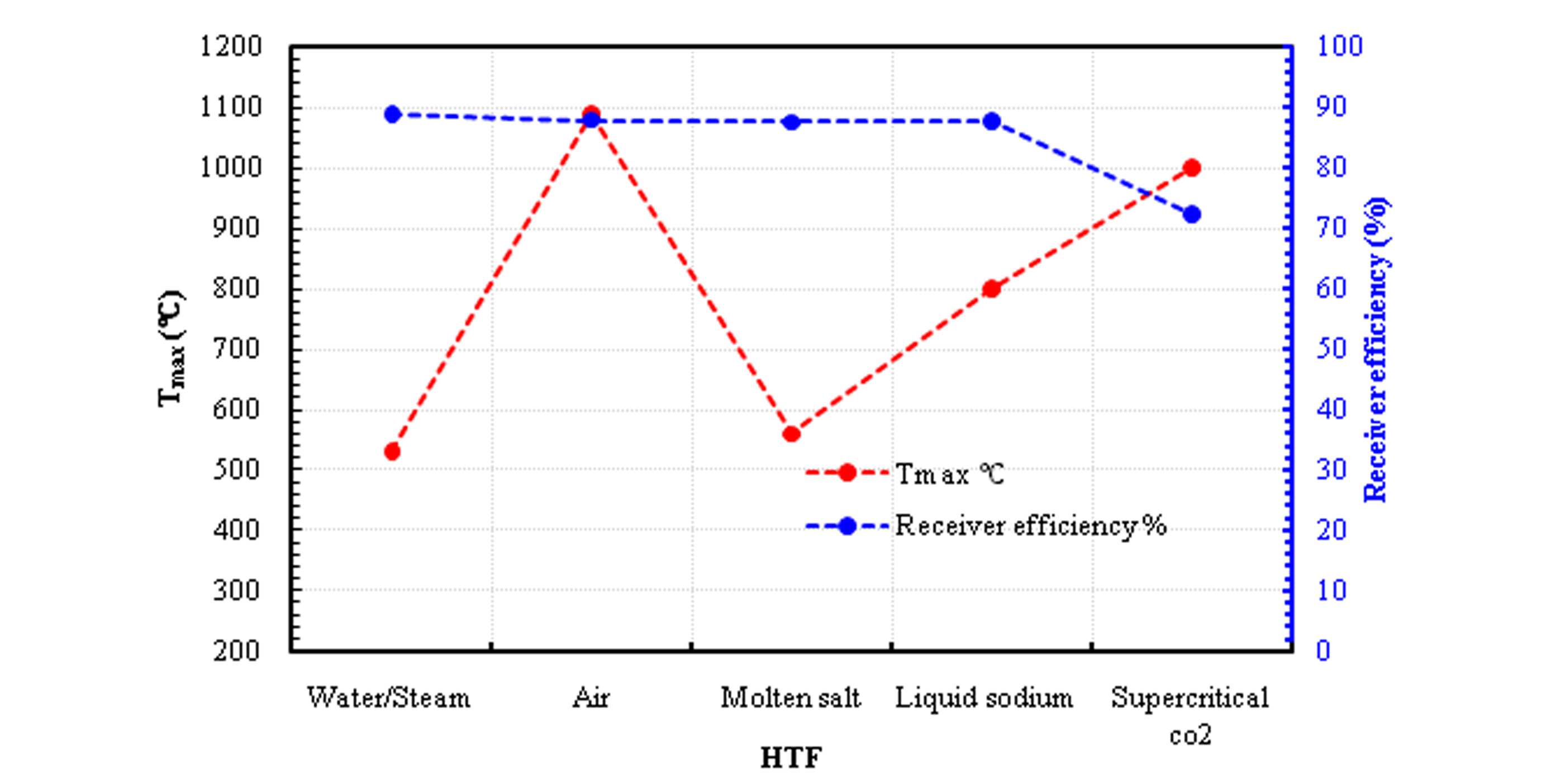
##submission.downloads##
Опубліковано
Як цитувати
Номер
Розділ
Ліцензія
Авторське право (c) 2022 Farah M. Falahat, Mohamed R. Gomaa

Ця робота ліцензується відповідно до Creative Commons Attribution 4.0 International License.
Закріплення та умови передачі авторських прав (ідентифікація авторства) здійснюється у Ліцензійному договорі. Зокрема, автори залишають за собою право на авторство свого рукопису та передають журналу право першої публікації цієї роботи на умовах ліцензії Creative Commons CC BY. При цьому вони мають право укладати самостійно додаткові угоди, що стосуються неексклюзивного поширення роботи у тому вигляді, в якому вона була опублікована цим журналом, але за умови збереження посилання на першу публікацію статті в цьому журналі.