Синтез та прогнозування ноотропної активності деяких похідних 4-(амінометил)-1-бензилпіролідин-2-ону, структурно споріднених з небрацетамом
DOI:
https://doi.org/10.15587/2519-4852.2024.310731Ключові слова:
синтез, 4-(амінометил)-1-бензилпіролідин-2-он, молекулярний докінг, ацетилхолінові рецептори, ноотропна активністьАнотація
Мета. Пошук нових біологічно активних речовин з покращеними ноотропними параметрами серед аналогів 4-(амінометил)-1-бензилпіролідин-2-ону (Небрацетаму).
Матеріали та методи. Необхідні реагенти очищали за допомогою стандартних методик. Елементний аналіз проводили на автоматичному аналізаторі М-180 фірми “HewlettPackard”. Спектри ЯМР 1Н записували на спектрометрі Varian Gemini 400 МГц у ДМСО-d6 як розчиннику. РХ/МС-спектри записували за допомогою рідинного хроматографа PE SCIEX API 150EX. Для молекулярного докінгу використовувався програмний пакет Autodock 4.2. Як біологічні мішені використовували активні центри пептидів (PDB ID: 5CXV, 6PV7).
Результати і обговорення. Для синтезу цільових аналогів 4-(амінометил)-1-R-бензилпіролідин-2-ону використано базовий та альтернативний методи (1 та 2) отримання. В результаті синтетичних досліджень запропоновано оптимізований метод з альтернативним шляхом, перевагами якого є скорочення тривалості та кількості стадій синтезу та уникнення використання азиду натрію, високотоксичної та небезпечної речовини. Молекулярний докінг синтезованих сполук на добре задокументованих сайтах рецепторів ацетилхоліну вказує на те, що всі протестовані молекули сприятимуть прояву ноотропної активності різним ступенем через механізми холінергічної нейротрансмісії. Про це свідчать розраховані значення докінгу по відношенню до мускаринової мішені. За результатами докінгу встановлено, що залежно від енантіомерної конфігурації молекули утворювали стійкі комплекси з мішенню та мали характерні режими зв’язування як в ортостеричному місці, так і в позаклітинному вестибюлі (місце позитивної алостеричної модуляції mAChR). Це вказує на перспективність модифікації «небрацетамового каркасу» за фенільним фрагментом галогенними замісниками.
Висновки. Розроблено ефективний метод синтезу аналогів 4-(амінометил)-1-R-бензилпіролідин-2-онів. Молекулярний докінг виявив потенційні механізми ноотропної дії синтезованих похідних як потенційних агоністів і позитивних алостеричних модуляторів мускаринового рецептора
Посилання
- Li Petri, G., Raimondi, M. V., Spanò, V., Holl, R., Barraja, P., Montalbano, A. (2021). Pyrrolidine in Drug Discovery: A Versatile Scaffold for Novel Biologically Active Compounds. Topics in Current Chemistry, 379 (5). https://doi.org/10.1007/s41061-021-00347-5
- Saiz Garcia, H., Montes Reula, L., Portilla Fernandez, A., Pereira Sanchez, V., Olmo Lopez, N., Mancha Heredero, E. et al. (2017). Nootropics: Emergents drugs associated with new clinical challenges. European Psychiatry, 41 (S1), s877–s878. https://doi.org/10.1016/j.eurpsy.2017.01.1769
- Alfaro-Rodríguez, A., Cortes-Altamirano, J., Olmos-Hernández, A., Bonilla-Jaime, H., Bandala, C., González-Maciel, A. (2016). Levetiracetam as an antiepileptic, neuroprotective, and hyperalgesic drug. Neurology India, 64 (6), 1266–1275. https://doi.org/10.4103/0028-3886.193801
- Urakami, K., Shimomura, T., Ohshima, T., Okada, A., Adachi, Y., Takahashi, K. et al. (1993). Clinical Effect of WEB 1881 (Nebracetam Fumarate) on Patients with Dementia of the Alzheimer Type and Study of Its Clinical Pharmacology. Clinical Neuropharmacology, 16 (4), 347–358. https://doi.org/10.1097/00002826-199308000-00007
- Kitamura, Y., Kaneda, T., Nomura, Y. (1991). Effects of nebracetam (WEB 1881 FU), a novel nootropic, as a M1-muscarinic agonist. The Japanese Journal of Pharmacology, 55 (1), 177–180. https://doi.org/10.1254/jjp.55.177
- Sahakian, B. J., Morein-Zamir, S. (2015). Pharmacological cognitive enhancement: treatment of neuropsychiatric disorders and lifestyle use by healthy people. The Lancet Psychiatry, 2 (4), 357–362. https://doi.org/10.1016/s2215-0366(15)00004-8
- Levin, E. D., Sledge, D., Roach, S., Petro, A., Donerly, S., Linney, E. (2011). Persistent behavioral impairment caused by embryonic methylphenidate exposure in zebrafish. Neurotoxicology and Teratology, 33 (6), 668–673. https://doi.org/10.1016/j.ntt.2011.06.004
- Battleday, R. M., Brem, A.-K. (2015). Modafinil for cognitive neuroenhancement in healthy non-sleep-deprived subjects: A systematic review. European Neuropsychopharmacology, 25 (11), 1865–1881. https://doi.org/10.1016/j.euroneuro.2015.07.028
- Vyas, S., Kothari, S. L., Kachhwaha, S. (2019). Nootropic medicinal plants: Therapeutic alternatives for Alzheimer’s disease. Journal of Herbal Medicine, 17-18, 100291. https://doi.org/10.1016/j.hermed.2019.100291
- Richter, N., Allendorf, I., Onur, O. A., Kracht, L., Dietlein, M., Tittgemeyer, M. et al. (2014). The integrity of the cholinergic system determines memory performance in healthy elderly. NeuroImage, 100, 481–488. https://doi.org/10.1016/j.neuroimage.2014.06.031
- Malykh, A. G., Sadaie, M. R. (2010). Piracetam and piracetam-like drugs: from basic science to novel clinical applications to CNS disorders. Drugs, 70 (3), 287–312. https://doi.org/10.2165/11319230-000000000-00000
- Uniyal, A., Singh, R., Akhtar, A., Bansal, Y., Kuhad, A., Sah, S. P. (2019). Co-treatment of piracetam with risperidone rescued extinction deficits in experimental paradigms of post-traumatic stress disorder by restoring the physiological alterations in cortex and hippocampus. Pharmacology Biochemistry and Behavior, 185, 172763. https://doi.org/10.1016/j.pbb.2019.172763
- Grossman, L., Stewart, A., Gaikwad, S., Utterback, E., Wu, N., DiLeo, J. et al. (2011). Effects of piracetam on behavior and memory in adult zebrafish. Brain Research Bulletin, 85(1–2), 58–63. https://doi.org/10.1016/j.brainresbull.2011.02.008
- Krintel, C., Harpsøe, K., Zachariassen, L. G., Peters, D., Frydenvang, K., Pickering, D. S. et al. (2013). Structural analysis of the positive AMPA receptor modulators CX516 and Me-CX516 in complex with the GluA2 ligand-binding domain. Acta Crystallographica Section D Biological Crystallography, 69 (9), 1645–1652. https://doi.org/10.1107/s0907444913011839
- Pugsley, T. A., Shih, Y., Coughenour, L., Stewart, S. F. (1983). Some neurochemical properties of pramiracetam (CI‐879), a new cognition‐enhancing agent. Drug Development Research, 3 (5), 407–420. https://doi.org/10.1002/ddr.430030503
- Nakashima, M. N., Kataoka, Y., Yamashita, K., Kohzuma, M., Ichikawa, M., Niwa, M. et al. (1995). Histological Evidence for Neuroprotective Action of Nebracetam on Ischemic Neuronal Injury in the Hippocampus of Stroke-Prone Spontaneously Hypertensive Rats. Japanese Journal of Pharmacology, 67 (1), 91–94. https://doi.org/10.1254/jjp.67.91
- Iwasaki, K., Matsumoto, Y., Fujiwara, M. (1992). Effect of Nebracetam on the Disruption of Spatial Cognition in Rats. The Japanese Journal of Pharmacology, 58 (2), 117–126. https://doi.org/10.1254/jjp.58.117
- Semenets, A. P., Suleiman, M. M., Fedosov, A. I., Shtrygol, S. Y., Havrylov, I. O., Mishchenko, M. V. et al. (2022). Synthesis, docking, and biological evaluation of novel 1-benzyl-4-(4-(R)-5-sulfonylidene-4,5-dihydro-1H-1,2,4-triazol-3-yl)pyrrolidin-2-ones as potential nootropic agents. European Journal of Medicinal Chemistry, 244, 114823. https://doi.org/10.1016/j.ejmech.2022.114823
- Semenets, A., Suleiman, M., Georgiyants, V., Kovalenko, S., Kobzar, N., Grinevich, L. et al. (2020). Theoretical justification of a purposeful search of potential neurotropic drugs. ScienceRise: Pharmaceutical Science, 4 (26), 4–17. https://doi.org/10.15587/2519-4852.2020.210042
- Yamashita, S., Mase, N., Takabe, K. (2008). Chemoenzymatic total synthesis and determination of the absolute configuration of (S)-nebracetam. Tetrahedron: Asymmetry, 19 (18), 2115–2118. https://doi.org/10.1016/j.tetasy.2008.09.004
- Gharpure, A., Teng, J., Zhuang, Y., Noviello, C. M., Walsh, R. M., Cabuco, R. et al. (2019). Agonist Selectivity and Ion Permeation in the α3β4 Ganglionic Nicotinic Receptor. Neuron, 104 (3), 501-511.e6. https://doi.org/10.1016/j.neuron.2019.07.030
- Grady, S. R., Moretti, M., Zoli, M., Marks, M. J., Zanardi, A., Pucci, L. et al (2009). Rodent Habenulo–Interpeduncular Pathway Expresses a Large Variety of Uncommon nAChR Subtypes, But Only the α3β4 and α3β3β4 Subtypes Mediate Acetylcholine Release. The Journal of Neuroscience, 29 (7), 2272–2282. https://doi.org/10.1523/jneurosci.5121-08.2009
- Albuquerque, E. X., Pereira, E. F. R., Alkondon, M., Rogers, S. W. (2009). Mammalian Nicotinic Acetylcholine Receptors: From Structure to Function. Physiological Reviews, 89 (1), 73–120. https://doi.org/10.1152/physrev.00015.2008
- Changeux, J.-P. (2018). The nicotinic acetylcholine receptor: a typical ‘allosteric machine.’ Philosophical Transactions of the Royal Society B: Biological Sciences, 373 (1749), 20170174. https://doi.org/10.1098/rstb.2017.0174
- Park, Y.-S., Kim, J., Kim, S.-H., Moon, Y.-J., Kwon, H.-M., Park, H.-S. et al. (2019). Comparison of recovery profiles in patients with Parkinson’s disease for 2 types of neuromuscular blockade reversal agent following deep brain stimulator implantation. Medicine, 98 (52), e18406. https://doi.org/10.1097/md.0000000000018406
- Thal, D. M., Sun, B., Feng, D., Nawaratne, V., Leach, K., Felder, C. C. et al. (2016). Crystal structures of the M1 and M4 muscarinic acetylcholine receptors. Nature, 531 (7594), 335–340. https://doi.org/10.1038/nature17188
- Kruse, A. C., Kobilka, B. K., Gautam, D., Sexton, P. M., Christopoulos, A., Wess, J. (2014). Muscarinic acetylcholine receptors: novel opportunities for drug development. Nature Reviews Drug Discovery, 13 (7), 549–560. https://doi.org/10.1038/nrd4295
- Changeux, J.-P. (2013). The concept of allosteric modulation: an overview. Drug Discovery Today: Technologies, 10 (2), e223–e228. https://doi.org/10.1016/j.ddtec.2012.07.007
- Dhama, N., Sucheta, Kumar, A., Verma, V., Kumar, S. (2021). A Review on Synthesis and Pharmacological Activities of Piracetam and its Derivatives. Asian Journal of Chemistry, 34 (1), 1–8. https://doi.org/10.14233/ajchem.2022.23357
- Jean, L., Baglin, I., Rouden, J., Maddaluno, J., Lasne, M.-C. (2001). A convenient route to 1-benzyl 3-aminopyrrolidine and 3-aminopiperidine. Tetrahedron Letters, 42 (33), 5645–5649. https://doi.org/10.1016/s0040-4039(01)00985-6
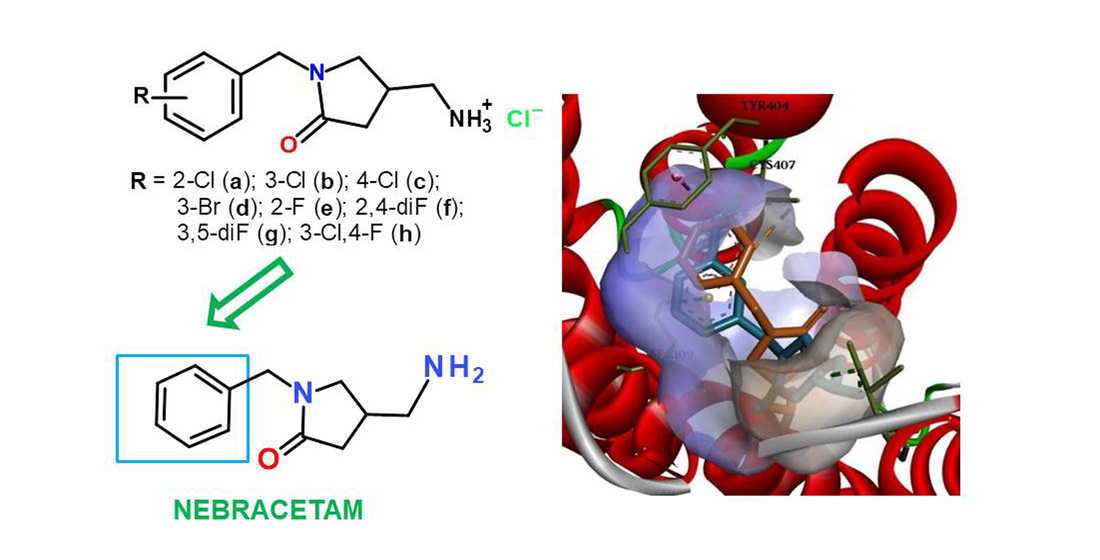
##submission.downloads##
Опубліковано
Як цитувати
Номер
Розділ
Ліцензія
Авторське право (c) 2024 Lina Perekhoda, Marharyta Suleiman, Illya Podolsky, Anton Semenets, Natalia Kobzar, Vitaliy Yaremenko, Olha Vislous, Victoriya Georgiyants, Sergiy Kovalenko

Ця робота ліцензується відповідно до Creative Commons Attribution 4.0 International License.
Наше видання використовує положення про авторські права Creative Commons CC BY для журналів відкритого доступу.