The application of arbutin in elimination resistance gramm-negative multidrug resistance bacteria of Pseudomonas aeruginosa and Enterobacter cloacea
DOI:
https://doi.org/10.15587/2519-4852.2024.305965Keywords:
arbutin, multi-drug resistant, Gram-negative strains, molecular docking, removal resistance, antibioticsAbstract
The basic therapy against microbial infections is the application of antibiotics. However, overuse of antibiotics has become the major factor for the emergence and dissemination of multidrug-resistant strains.
The aim. The purpose of our work was to investigate the in vitro and in silico elimination resistance of antibiotics (сlarithromycin, azithromycin, gentamycin, ciprofloxacin, levofloxacin, ceftriaxone, and chloramphenicol) against clinical multidrug-resistant strains of P. aeruginosa and E. cloacae by arbutin.
Materials and methods. The molecular docking was performed using AutoDockTools 1.5.6; antimicrobial effects were evaluated by the well method. Isolates were obtained from clinical samples including tracheal aspirate and broncoalveolar lavage.
Results. Theoretical studies have found that none of the investigated antibiotics and arbutin highly selectively inhibit all "targets" mechanisms of antimicrobial action. In experimental studies, it was observed that adding arbutin to the antibiotic led to the emergence of sensitivity on the part of resistant strains. All Gramm-negative resistance strains of bacteria were sensitive to the action of arbutin. Moreover, arbutin increased the antimicrobial effect of antibiotics from 8 to 55 %. It was estimated exceptions such as clarithromycin and azithromycin when assessing antimicrobial activity against P. aeruginosa.
Conclusions. These studies have shown that inhibiting resistant strains of bacteria requires the use of combinations of "classical" antimicrobials and herbal drugs or dietary supplements based on extracts obtained from arbutin-containing medicinal plants such as lingonberry, bearberry, and cranberry. This approach is a “lifeline” for the development of antimicrobial agents against resistant bacteria and gives “a second chance to return to life” for outdated antibiotics
Supporting Agency
- Ministry of Health of Ukraine, carried out at the expense of the state budget of Ukraine No. 0124U002080 (Order of the Ministry of Health of Ukraine No. 17 of 27.02.2024). We are grateful for the scientific and material help provided by the pharmaceutical company "Astrapharm" Kyiv, Ukraine, and the pharmaceutical company "Zdravopharm", Kharkiv, Ukraine.
References
- Mende, K., Akers, K. S., Tyner, S. D., Bennett, J. W., Simons, M. P., Blyth, D. M. et al. (2022). Multidrug-Resistant and Virulent Organisms Trauma Infections: Trauma Infectious Disease Outcomes Study Initiative. Military Medicine, 187 (Supplement_2), 42–51. https://doi.org/10.1093/milmed/usab131
- Kondratiuk, V., Jones, B. T., Kovalchuk, V., Kovalenko, I., Ganiuk, V., Kondratiuk, O., Frantsishko, A. (2021). Phenotypic and genotypic characterization of antibiotic resistance in military hospital-associated bacteria from war injuries in the Eastern Ukraine conflict between 2014 and 2020. Journal of Hospital Infection, 112, 69–76. https://doi.org/10.1016/j.jhin.2021.03.020
- Petrosillo, N., Petersen, E., Antoniak, S. (2023). Ukraine war and antimicrobial resistance. The Lancet Infectious Diseases, 23 (6), 653–654. https://doi.org/10.1016/s1473-3099(23)00264-5
- Antimicrobial resistance surveillance in Europe 2022. 2020 data (2022). WHO Regional Office for Europe (WHO/Europe)/European Centre for Disease Prevention and Control (ECDC). Copenhagen: WHO/Europe. Available at: https://www.ecdc.europa.eu/sites/default/files/documents/ECDC-WHO-AMR-report.pdf
- Schultze, T., Hogardt, M., Velázquez, E. S., Hack, D., Besier, S., Wichelhaus, T. A. et al. (2023). Molecular surveillance of multidrug-resistant Gram-negative bacteria in Ukrainian patients, Germany, March to June 2022. Eurosurveillance, 28 (1). https://doi.org/10.2807/1560-7917.es.2023.28.1.2200850
- Maslov, O., Komisarenko, M., Kolisnyk, S., Tkachenko, O., Akhmedov, E., Poluain, S. et al. (2023). Study of qualitative composition and quantitative content of free organic acids in lingberry leaves. Fitoterapia, 1, 77–82. https://doi.org/10.32782/2522-9680-2023-1-77
- Zhou, H., Zhao, J., Li, A., Reetz, M. T. (2019). Chemical and Biocatalytic Routes to Arbutin †. Molecules, 24 (18), 3303. https://doi.org/10.3390/molecules24183303
- Ma, C., He, N., Zhao, Y., Xia, D., Wei, J., Kang, W. (2019). Antimicrobial Mechanism of Hydroquinone. Applied Biochemistry and Biotechnology, 189 (4), 1291–1303. https://doi.org/10.1007/s12010-019-03067-1
- Maslov, O., Komisarenko, M., Ponomarenko, S., Horopashna, D., Osolodchenko, T., Kolisnyk, S. et al. (2022). Investigation the influence of biologically active compounds on the antioxidant, antibacterial and anti-inflammatory activities of red raspberry (Rubus idaeous l.) leaf extract. Current Issues in Pharmacy and Medical Sciences, 35 (4), 229–235. https://doi.org/10.2478/cipms-2022-0040
- Osolodchenko, T., Andreieva, I., Martynov, A., Nadiya, Z., Batrak, O., Ryabova, I. (2024). Monitoring of time stability of antimicrobial effect of nisin-based pharmaceutical compositions with resistance inhibitors. Annals of Mechnikov Institute, 3, 40–46. https://doi.org/10.5281/zenodo.13819951
- Morris, G. M., Huey, R., Olson, A. J. (2008). Using AutoDock for Ligand‐Receptor Docking. Current Protocols in Bioinformatics, 24 (1). https://doi.org/10.1002/0471250953.bi0814s24
- RCSB PDB: Homepage. RCSB PDB: Homepage. Available at: https://www.rcsb.org/
- PubChem. Available at: https://pubchem.ncbi.nlm.nih.gov/
- CASTp 3.0: Computed Atlas of Surface Topography of proteins. Available at: http://sts.bioe.uic.edu/castp/index.html?201l
- Kondža, M., Brizić, I., Jokić, S. (2024). Flavonoids as CYP3A4 Inhibitors In Vitro. Biomedicines, 12 (3), 644. https://doi.org/10.3390/biomedicines12030644
- Pulingam, T., Parumasivam, T., Gazzali, A. M., Sulaiman, A. M., Chee, J. Y., Lakshmanan, M. et al. (2022). Antimicrobial resistance: Prevalence, economic burden, mechanisms of resistance and strategies to overcome. European Journal of Pharmaceutical Sciences, 170, 106103. https://doi.org/10.1016/j.ejps.2021.106103
- Abinaya, M., Gayathri, M. (2019). Inhibition of biofilm formation, quorum sensing activity and molecular docking study of isolated 3, 5, 7-Trihydroxyflavone from Alstonia scholaris leaf against P.aeruginosa. Bioorganic Chemistry, 87, 291–301. https://doi.org/10.1016/j.bioorg.2019.03.050
- Jogula, S., Krishna, V. S., Meda, N., Balraju, V., Sriram, D. (2020). Design, synthesis and biological evaluation of novel Pseudomonas aeruginosa DNA gyrase B inhibitors. Bioorganic Chemistry, 100, 103905. https://doi.org/10.1016/j.bioorg.2020.103905
- Mbarga, M. J. A., Podoprigora, I. V., Volina, E. G., Ermolaev, A. V., Smolyakova, L. A. (2021). Evaluation of Changes Induced in the Probiotic Escherichia coli M17 Following Recurrent Exposure to Antimicrobials. Journal of Pharmaceutical Research International, 158–167. https://doi.org/10.9734/jpri/2021/v33i29b31601
- Zuo, K., Liang, L., Du, W., Sun, X., Liu, W., Gou, X. et al. (2017). 3D-QSAR, Molecular Docking and Molecular Dynamics Simulation of Pseudomonas aeruginosa LpxC Inhibitors. International Journal of Molecular Sciences, 18 (5), 761. https://doi.org/10.3390/ijms18050761
- Abinaya, M., Gayathri, M. (2019). Inhibition of biofilm formation, quorum sensing activity and molecular docking study of isolated 3, 5, 7-Trihydroxyflavone from Alstonia scholaris leaf against P.aeruginosa. Bioorganic Chemistry, 87, 291–301. https://doi.org/10.1016/j.bioorg.2019.03.050
- Jean, S.-S., Harnod, D., Hsueh, P.-R. (2022). Global Threat of Carbapenem-Resistant Gram-Negative Bacteria. Frontiers in Cellular and Infection Microbiology, 12. https://doi.org/10.3389/fcimb.2022.823684
- Aranaga, C., Pantoja, L. D., Martínez, E. A., Falco, A. (2022). Phage Therapy in the Era of Multidrug Resistance in Bacteria: A Systematic Review. International Journal of Molecular Sciences, 23 (9), 4577. https://doi.org/10.3390/ijms23094577
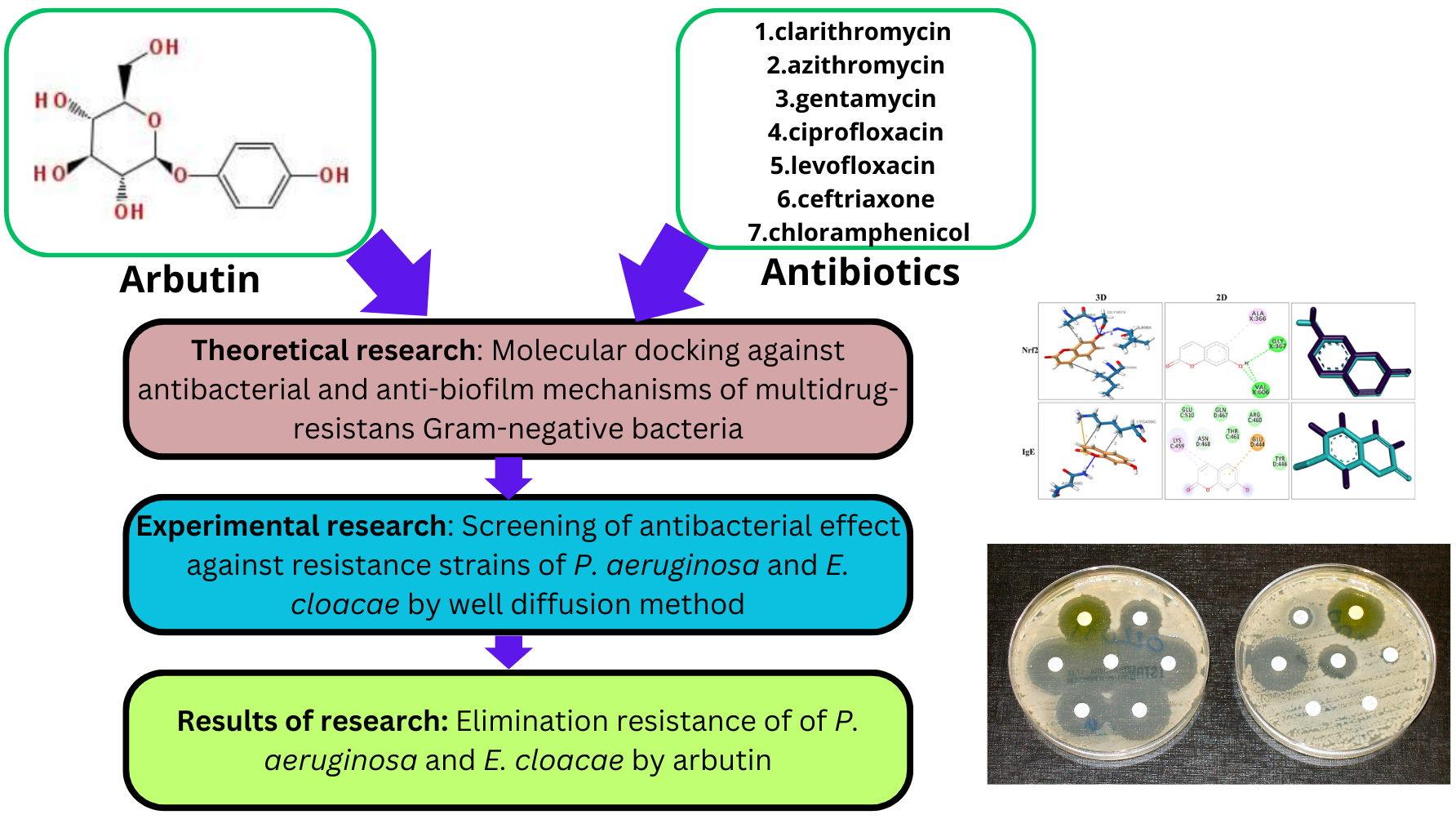
Downloads
Published
How to Cite
Issue
Section
License
Copyright (c) 2024 Oleksandr Maslov, Mykola Komisarenko , Svitlana Ponomarenko, Tetiana Osolodchenko, Sergii Kolisnyk , Oleh Koshovyi, Andrey Komissarenko

This work is licensed under a Creative Commons Attribution 4.0 International License.
Our journal abides by the Creative Commons CC BY copyright rights and permissions for open access journals.