Neutronic design of small modular longlife pressurized water reactor using thorium carbide fuel at a power level of 300–500 MWth
DOI:
https://doi.org/10.15587/1729-4061.2024.290996Keywords:
thorium, core design, Doppler reactivity, void fraction coefficient, CITATIONAbstract
This study presents the neutronic design of a small modular longlife Pressurized Water Reactor (PWR) using thorium carbide fuel with 233U fissile material. The target optimization for this study is a reactor designed to operate for 20 years, with excess reactivity throughout the reactor operational cycle consistently below 1.00 % dk/k. The analysis involves dividing the reactor core into three fuel regions with 233U enrichment levels ranging from 3 % to 8 %, with a 1 % difference for each fuel region. To achieve optimum conditions, 231Pa was randomly added to the fuel. The fuel volume fraction in this design varied from 30 % to 65 %, with a 5 % incremental variation. Power level variations are also studied within the 300–500 MWth with increments of 50 MWth. Calculations were performed using the Standard Reactor Analysis Code (SRAC) program with the PIJ and CITATION modules for cell and core calculations utilizing JENDL4.0 nuclide data. Neutronic calculations indicate that the fuel with a 60 % volume fraction achieves optimum conditions at a power level of 300 MWth. The best performance was observed with a fuel volume fraction of 65 %, reaching optimum conditions across power levels ranging from 300 to 500 MWth. For the fuel with the best performance, the power density distributions for low and high power levels follow the same pattern radially and axially. The power peaking factor (PPF) for all fuel configurations approaching the optimum conditions remains below two, a safe limit for the PWR. Other neutronic safety parameters, such as the Doppler coefficient and void fraction coefficient, also stay within the safe limits for the PWR, with both values remaining negative throughout the reactor operational cycle
References
- Nuclear Power in the World Today. Available at: https://world-nuclear.org/information-library/current-and-future-generation/nuclear-power-in-the-world-today.aspx
- Cummins, W. E., Matzie, R. (2018). Design evolution of PWRs: Shippingport to generation III+. Progress in Nuclear Energy, 102, 9–37. https://doi.org/10.1016/j.pnucene.2017.08.008
- Morales Pedraza, J. (2017). Small Modular Reactors for Electricity Generation. Springer International Publishing. https://doi.org/10.1007/978-3-319-52216-6
- Mittag, S., Kliem, S. (2011). Burning plutonium and minimizing radioactive waste in existing PWRs. Annals of Nuclear Energy, 38 (1), 98–102. https://doi.org/10.1016/j.anucene.2010.08.012
- Li, J., Li, X., Cai, J. (2021). Neutronic characteristics and feasibility analysis of micro-heterogeneous duplex ThO2-UO2 fuel pin in PWR. Nuclear Engineering and Design, 382, 111382. https://doi.org/10.1016/j.nucengdes.2021.111382
- Galahom, A. A., Mohsen, M. Y. M., Amrani, N. (2022). Explore the possible advantages of using thorium-based fuel in a pressurized water reactor (PWR) Part 1: Neutronic analysis. Nuclear Engineering and Technology, 54 (1), 1–10. https://doi.org/10.1016/j.net.2021.07.019
- Mirvakili, S. M., Alizadeh Kavafshary, M., Joze Vaziri, A. (2015). Comparison of neutronic behavior of UO2 , (Th- 233 U)O 2 and (Th- 235 U)O2 fuels in a typical heavy water reactor. Nuclear Engineering and Technology, 47 (3), 315–322. https://doi.org/10.1016/j.net.2014.12.014
- Baldova, D., Fridman, E., Shwageraus, E. (2014). High conversion Th-U233 fuel for current generation of PWRs: Part I – Assembly level analysis. Annals of Nuclear Energy, 73, 552–559. https://doi.org/10.1016/j.anucene.2014.05.017
- Gorton, J. P., Collins, B. S., Nelson, A. T., Brown, N. R. (2019). Reactor performance and safety characteristics of ThN-UN fuel concepts in a PWR. Nuclear Engineering and Design, 355, 110317. https://doi.org/10.1016/j.nucengdes.2019.110317
- Mohsen, M. Y. M., Abdel-Rahman, M. A. E., Galahom, A. A. (2021). Ensuring the possibility of using thorium as a fuel in a pressurized water reactor (PWR). Nuclear Science and Techniques, 32 (12). https://doi.org/10.1007/s41365-021-00981-0
- Maiorino, J. R., Stefani, G. L., Moreira, J. M. L., Rossi, P. C. R., Santos, T. A. (2017). Feasibility to convert an advanced PWR from UO2 to a mixed U/ThO2 core – Part I: Parametric studies. Annals of Nuclear Energy, 102, 47–55. https://doi.org/10.1016/j.anucene.2016.12.010
- Akbari-Jeyhouni, R., Rezaei Ochbelagh, D., Maiorino, J. R., D’Auria, F., Stefani, G. L. de (2018). The utilization of thorium in Small Modular Reactors – Part I: Neutronic assessment. Annals of Nuclear Energy, 120, 422–430. https://doi.org/10.1016/j.anucene.2018.06.013
- Lau, C. W., Nylén, H., Demazière, C., Sandberg, U. (2014). Reducing axial offset and improving stability in PWRs by using uranium–thorium fuel. Progress in Nuclear Energy, 76, 137–147. https://doi.org/10.1016/j.pnucene.2014.05.016
- Vaidyanathan, S. (2021). Transitioning to a Sustainable Thorium Fuel Cycle in Pressurized Water Reactors Using Bimetallic Thorium-Zirconium Alloy Cladding. Nuclear Technology, 207 (12), 1793–1809. https://doi.org/10.1080/00295450.2020.1846987
- Peakman, A., Owen, H., Abram, T. (2021). Core design and fuel behaviour of a small modular pressurised water reactor using (Th,U)O2 fuel for commercial marine propulsion. Progress in Nuclear Energy. https://doi.org/10.1016/j.pnucene.2021.103966
- Subkhi, M. N., Su’ud, Z., Waris, A. (2013). Netronic Design of Small Long-Life PWR Using Thorium Cycle. Advanced Materials Research, 772, 524–529. https://doi.org/10.4028/www.scientific.net/amr.772.524
- Syarifah, R. D., Aula, M. H., Ardianingrum, A., Janah, L. N., Maulina, W. (2022). Comparison of thorium nitride and uranium nitride fuel on small modular pressurized water reactor in neutronic analysis using SRAC code. Eastern-European Journal of Enterprise Technologies, 2 (8 (116)), 21–28. https://doi.org/10.15587/1729-4061.2022.255849
- Kim, T. K., Grandy, C., Hill, R. N. (2009). Carbide and Nitride Fuels for Advanced Burner Reactor. International Conference on Fast Reactors and Related Fuel Cycles (FR09) - Challenges and Opportunities. Available at: https://inis.iaea.org/collection/NCLCollectionStore/_Public/41/070/41070109.pdf
- Lapanporo, B. P., Su’ud, Z. (2022). Parametric Study of Thorium Fuel Utilization on Small Modular Pressurized Water Reactors (PWR). Journal of Physics: Conference Series, 2243 (1), 012062. https://doi.org/10.1088/1742-6596/2243/1/012062
- Subki, I., Pramutadi, A., Rida, S. N. M., Su’ud, Z., Eka Sapta, R., Muh. Nurul, S. et al. (2008). The utilization of thorium for long-life small thermal reactors without on-site refueling. Progress in Nuclear Energy, 50 (2-6), 152–156. https://doi.org/10.1016/j.pnucene.2007.10.029
- Dobuchi, N., Takeda, S., Kitada, T. (2016). Study on the relation between Doppler reactivity coefficient and resonance integrals of Thorium and Uranium in PWR fuels. Annals of Nuclear Energy, 90, 191–194. https://doi.org/10.1016/j.anucene.2015.11.018
- Functional Design of Reactivity Control Systems. AP1000 Design Control Document. Available at: https://www.nrc.gov/docs/ML1117/ML11171A448.pdf
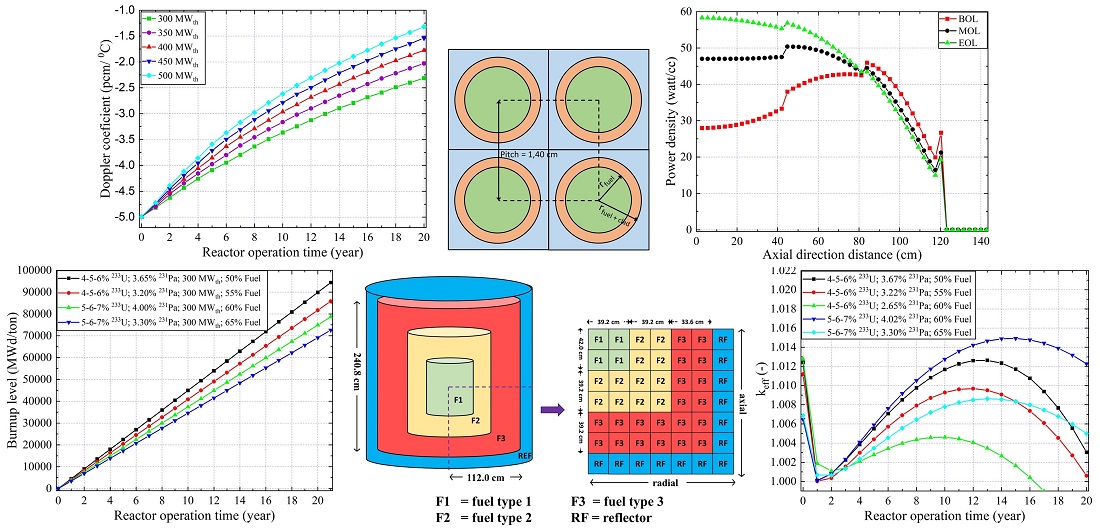
Downloads
Published
How to Cite
Issue
Section
License
Copyright (c) 2024 Boni Pahlanop Lapanporo, Zaki Su’ud, Asril Pramutadi Andi Mustari

This work is licensed under a Creative Commons Attribution 4.0 International License.
The consolidation and conditions for the transfer of copyright (identification of authorship) is carried out in the License Agreement. In particular, the authors reserve the right to the authorship of their manuscript and transfer the first publication of this work to the journal under the terms of the Creative Commons CC BY license. At the same time, they have the right to conclude on their own additional agreements concerning the non-exclusive distribution of the work in the form in which it was published by this journal, but provided that the link to the first publication of the article in this journal is preserved.
A license agreement is a document in which the author warrants that he/she owns all copyright for the work (manuscript, article, etc.).
The authors, signing the License Agreement with TECHNOLOGY CENTER PC, have all rights to the further use of their work, provided that they link to our edition in which the work was published.
According to the terms of the License Agreement, the Publisher TECHNOLOGY CENTER PC does not take away your copyrights and receives permission from the authors to use and dissemination of the publication through the world's scientific resources (own electronic resources, scientometric databases, repositories, libraries, etc.).
In the absence of a signed License Agreement or in the absence of this agreement of identifiers allowing to identify the identity of the author, the editors have no right to work with the manuscript.
It is important to remember that there is another type of agreement between authors and publishers – when copyright is transferred from the authors to the publisher. In this case, the authors lose ownership of their work and may not use it in any way.