Enhancing savonius rotor model with additional grooves on hydrokinetic turbine performance
DOI:
https://doi.org/10.15587/1729-4061.2024.298915Keywords:
hydrokinetic turbine, Savonius rotor, grooved blade, drag coefficient, tip speed ratioAbstract
Hydrokinetic turbines use different rotors for technological and economic reasons. Even though it performs poorly, vertical-axis hydrokinetic turbines use the Savonius rotor. The object of research is a Savonius rotor model with additional grooves. The study addresses the need to improve the efficiency and overall performance of Savonius rotor models in hydrokinetic turbines, which are widely used for harnessing energy from flowing water currents. The problem involves understanding how different groove configurations affect the aerodynamic behavior and energy extraction efficiency of the Savonius rotor in hydrokinetic turbine applications. The test results revealed that incorporating grooves led to notable improvements in efficiency (ɳ) and coefficient of drag (CD). Grooved blades exhibited a maximum efficiency of 30.97 % and a maximum drag coefficient of 2.71. Notably, blades with a groove width of 12.5 mm emerged as the optimal model, demonstrating an efficiency peak of 35.66 % and a drag coefficient 3.08. This indicates a substantial increase in efficiency by 4.69 % and a corresponding rise in the drag coefficient by 0.37 for grooved blades. The grooves on grooved blades increase friction, improving performance. Grooved rotor blades improve turbine performance significantly. Savonius rotor models in hydrokinetic turbines extract more energy by optimizing groove width and arrangement to maximize drag coefficient and efficiency. This research affects hydrokinetic turbine design and optimization for renewable energy generation. Engineers and designers can improve the performance and efficiency of the Savonius rotor model in hydrokinetic turbine applications by applying this study’s findings
Supporting Agency
- The author would like to extend their deepest appreciation to the Mechanical Engineering Department at Hasanuddin University in South Sulawesi for their help and support in publishing this study.
References
- Yuce, M. I., Muratoglu, A. (2015). Hydrokinetic energy conversion systems: A technology status review. Renewable and Sustainable Energy Reviews, 43, 72–82. https://doi.org/10.1016/j.rser.2014.10.037
- Maldar, N. R., Ng, C. Y., Oguz, E. (2020). A review of the optimization studies for Savonius turbine considering hydrokinetic applications. Energy Conversion and Management, 226, 113495. https://doi.org/10.1016/j.enconman.2020.113495
- Sule, L., Mochtar, A. A., Sutresman, O. (2020). Performance of Undershot Water Wheel with Bowl-shaped Blades Model. International Journal of Technology, 11 (2), 278. https://doi.org/10.14716/ijtech.v11i2.2465
- Talukdar, P. K., Sardar, A., Kulkarni, V., Saha, U. K. (2018). Parametric analysis of model Savonius hydrokinetic turbines through experimental and computational investigations. Energy Conversion and Management, 158, 36–49. https://doi.org/10.1016/j.enconman.2017.12.011
- Zhang, Y., Kang, C., Ji, Y., Li, Q. (2019). Experimental and numerical investigation of flow patterns and performance of a modified Savonius hydrokinetic rotor. Renewable Energy, 141, 1067–1079. https://doi.org/10.1016/j.renene.2019.04.071
- Kumar, A., Saini, R. P. (2017). Performance analysis of a single stage modified Savonius hydrokinetic turbine having twisted blades. Renewable Energy, 113, 461–478. https://doi.org/10.1016/j.renene.2017.06.020
- Basumatary, M., Biswas, A., Misra, R. D. (2018). CFD analysis of an innovative combined lift and drag (CLD) based modified Savonius water turbine. Energy Conversion and Management, 174, 72–87. https://doi.org/10.1016/j.enconman.2018.08.025
- Alizadeh, H., Jahangir, M. H., Ghasempour, R. (2020). CFD-based improvement of Savonius type hydrokinetic turbine using optimized barrier at the low-speed flows. Ocean Engineering, 202, 107178. https://doi.org/10.1016/j.oceaneng.2020.107178
- Sarma, N. K., Biswas, A., Misra, R. D. (2014). Experimental and computational evaluation of Savonius hydrokinetic turbine for low velocity condition with comparison to Savonius wind turbine at the same input power. Energy Conversion and Management, 83, 88–98. https://doi.org/10.1016/j.enconman.2014.03.070
- Tian, W., Mao, Z., Ding, H. (2018). Design, test and numerical simulation of a low-speed horizontal axis hydrokinetic turbine. International Journal of Naval Architecture and Ocean Engineering, 10 (6), 782–793. https://doi.org/10.1016/j.ijnaoe.2017.10.006
- Zahariev, M. E. (2016). Flow diagnostics and optimal design of vertical axis wind turbines for urban environments. University of Huddersfield. Available at: https://eprints.hud.ac.uk/id/eprint/31542/
- Salam, N., Tarakka, R., Jalaluddin, Jimran, M. A., Ihsan, M. (2021). Flow Separation in Four Configurations of Three Tandem Minibus Models. International Journal of Mechanical Engineering and Robotics Research, 10 (5), 236–247. https://doi.org/10.18178/ijmerr.10.5.236-247
- Torres, S., Marulanda, A., Montoya, M. F., Hernandez, C. (2022). Geometric design optimization of a Savonius wind turbine. Energy Conversion and Management, 262, 115679. https://doi.org/10.1016/j.enconman.2022.115679
- Sharma, S., Sharma, R. K. (2016). Performance improvement of Savonius rotor using multiple quarter blades – A CFD investigation. Energy Conversion and Management, 127, 43–54. https://doi.org/10.1016/j.enconman.2016.08.087
- Kerikous, E., Thévenin, D. (2019). Optimal shape of thick blades for a hydraulic Savonius turbine. Renewable Energy, 134, 629–638. https://doi.org/10.1016/j.renene.2018.11.037
- Sodjavi, K., Ravelet, F., Bakir, F. (2018). Effects of axial rectangular groove on turbulent Taylor-Couette flow from analysis of experimental data. Experimental Thermal and Fluid Science, 97, 270–278. https://doi.org/10.1016/j.expthermflusci.2018.04.022
- Kerikous, E., Thévenin, D. (2019). Performance Enhancement of a Hydraulic Savonius Turbine by Optimizing Overlap and Gap Ratios. Volume 2: Combustion, Fuels, and Emissions; Renewable Energy: Solar and Wind; Inlets and Exhausts; Emerging Technologies: Hybrid Electric Propulsion and Alternate Power Generation; GT Operation and Maintenance; Materials and Manufacturing (Including Coatings, Composites, CMCs, Additive Manufacturing); Analytics and Digital Solutions for Gas Turbines/Rotating Machinery. https://doi.org/10.1115/gtindia2019-2670
- Soenoko, R., Purnami, P. (2019). Bowl bladed hydrokinetic turbine with additional steering blade numerical modeling. Eastern-European Journal of Enterprise Technologies, 4 (8 (100)), 24–36. https://doi.org/10.15587/1729-4061.2019.173986
- Cengel, Y., Cimbala, J. (2013). Fluid mechanics fundamentals and applications. McGraw Hill, 1000.
- Kini, C. R., Sharma, N. Y., Shenoy B., S. (2017). Fluid Structure Interaction Study of High Pressure Stage Gas Turbine Blade Having Grooved Cooling Channels. International Review of Mechanical Engineering (IREME), 11 (11), 825. https://doi.org/10.15866/ireme.v11i11.12465
- Ahmadi-Baloutaki, M., Carriveau, R., Ting, D. S.-K. (2013). Effect of free-stream turbulence on flow characteristics over a transversely-grooved surface. Experimental Thermal and Fluid Science, 51, 56–70. https://doi.org/10.1016/j.expthermflusci.2013.07.001
- Wan Yahaya, W. M. A., Samion, S., Mohd Zawawi, F., Musa, M. N., Najurudeen, M. N. A. (2020). The Evaluation of Drag and Lift Force of Groove Cylinder in Wind Tunnel. Journal of Advanced Research in Fluid Mechanics and Thermal Sciences, 68 (2), 41–50. https://doi.org/10.37934/arfmts.68.2.4150
- Gowree, E. R., Jagadeesh, C., Atkin, C. J. (2019). Skin friction drag reduction over staggered three dimensional cavities. Aerospace Science and Technology, 84, 520–529. https://doi.org/10.1016/j.ast.2018.11.001
- Seo, S.-H., Hong, C.-H. (2015). Performance improvement of airfoils for wind blade with the groove. International Journal of Green Energy, 13 (1), 34–39. https://doi.org/10.1080/15435075.2014.910777
- Yao, J., Teo, C. J. (2022). Drag reduction by a superhydrophobic surface with longitudinal grooves: the effects of the rib surface curvature. Journal of Turbulence, 23 (8), 405–432. https://doi.org/10.1080/14685248.2022.2094936
- Chehouri, A., Younes, R., Ilinca, A., Perron, J. (2015). Review of performance optimization techniques applied to wind turbines. Applied Energy, 142, 361–388. https://doi.org/10.1016/j.apenergy.2014.12.043
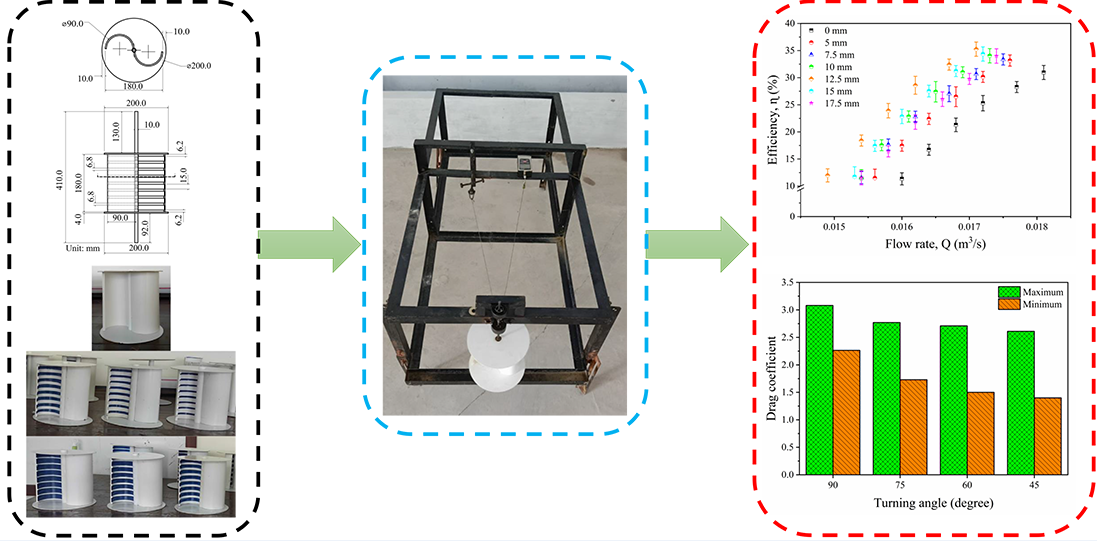
Downloads
Published
How to Cite
Issue
Section
License
Copyright (c) 2024 Petrus Sampelawang, Nasaruddin Salam, Luther Sule, Rustan Tarakka

This work is licensed under a Creative Commons Attribution 4.0 International License.
The consolidation and conditions for the transfer of copyright (identification of authorship) is carried out in the License Agreement. In particular, the authors reserve the right to the authorship of their manuscript and transfer the first publication of this work to the journal under the terms of the Creative Commons CC BY license. At the same time, they have the right to conclude on their own additional agreements concerning the non-exclusive distribution of the work in the form in which it was published by this journal, but provided that the link to the first publication of the article in this journal is preserved.
A license agreement is a document in which the author warrants that he/she owns all copyright for the work (manuscript, article, etc.).
The authors, signing the License Agreement with TECHNOLOGY CENTER PC, have all rights to the further use of their work, provided that they link to our edition in which the work was published.
According to the terms of the License Agreement, the Publisher TECHNOLOGY CENTER PC does not take away your copyrights and receives permission from the authors to use and dissemination of the publication through the world's scientific resources (own electronic resources, scientometric databases, repositories, libraries, etc.).
In the absence of a signed License Agreement or in the absence of this agreement of identifiers allowing to identify the identity of the author, the editors have no right to work with the manuscript.
It is important to remember that there is another type of agreement between authors and publishers – when copyright is transferred from the authors to the publisher. In this case, the authors lose ownership of their work and may not use it in any way.