Optimization of the electrostatic coal adsorption process for sea-salt production from seawater
DOI:
https://doi.org/10.15587/1729-4061.2023.279099Keywords:
electrostatic coal adsorbent, sea-salt impurities, sulfate adsorption, lime juice, acid-washAbstract
High sulfate content in seawater forms sulfate salts, which become impurities in sea salts. This study investigates the influence of lime juice in the adsorption of sulfate ions in seawater using commercial activated carbon. A full factorial experimental design was employed to optimize the level factors of activated carbon type, adsorbent dosage, and concentration of lime juice in response to the percentage reduction in sulfate concentration. Activated carbon (GCB) and acid-washed activated carbon (GCA) were two types of coconut shells granular activated carbon used for the experiment without further modification. The main effect and interaction effects were analyzed using analysis of variance (ANOVA) and p-values to define the influence of variables affecting sulfate ions adsorption. The adsorption of sulfate ions in seawater was affected by the interaction between the activated carbon type and the dosage, and the concentration of lime juice. The lime juice factor significantly enhanced the performance of activated carbon to adsorb the sulfate ions in seawater, and the factor's contribution was 58.2 %. The optimum sulfate ions reduction from seawater was attained at levels of factors activated carbon GCB, the dosage of 50mg, and the concentration of lime juice 50 µl. The interaction between lime juice and activated carbon pores are electrostatic. The impurities are attracted by the revealed polarity of the activated carbon pores. High electronegativity of lime juice acid pulls the negatively charged ions of the impurities. The more economical activated carbon, GCB, which performed better in sulfate ion adsorption, provides an alternative for reducing sea salt impurities. Hence, GCB can directly be mixed with the seawater to produce high quality sea-salt. Therefore, this study is suitable to improve sea salt product quality that processed with activated carbon
Supporting Agency
- We would like to thank the support of the FESEM facility (FEI Quanta FEG 650) at Central Laboratory of Life Science-Brawijaya University (LSIH-UB)
References
- Dasgupta, P. K., Liu, Y., Dyke, J. V. (2008). Iodine Nutrition: Iodine Content of Iodized Salt in the United States. Environmental Science & Technology, 42 (4), 1315–1323. doi: https://doi.org/10.1021/es0719071
- Knowles, J. M., Garrett, G. S., Gorstein, J., Kupka, R., Situma, R., Yadav, K. et al. (2017). Household Coverage with Adequately Iodized Salt Varies Greatly between Countries and by Residence Type and Socioeconomic Status within Countries: Results from 10 National Coverage Surveys. The Journal of Nutrition, 147 (5), 1004S-1014S. doi: https://doi.org/10.3945/jn.116.242586
- Sedivy, V. M. (2013). Environmental balance of salt production speaks in favour of solar saltworks. Global NEST Journal, 11 (1), 41–48. doi: https://doi.org/10.30955/gnj.000567
- Byrne, R. H., Mackenzie, F. T., Duxbury, A. C. (2023). Seawater. Encyclopedia Britannica. Available at: https://www.britannica.com/science/seawater
- Keene, W. C., Pszenny, A. A. P., Galloway, J. N., Hawley, M. E. (1986). Sea-salt corrections and interpretation of constituent ratios in marine precipitation. Journal of Geophysical Research, 91 (D6), 6647. doi: https://doi.org/10.1029/jd091id06p06647
- Runtti, H., Tuomikoski, S., Kangas, T., Kuokkanen, T., Rämö, J., Lassi, U. (2016). Sulphate Removal from Water by Carbon Residue from Biomass Gasification: Effect of Chemical Modification Methods on Sulphate Removal Efficiency. BioResources, 11 (2). doi: https://doi.org/10.15376/biores.11.2.3136-3152
- Hong, S., Cannon, F. S., Hou, P., Byrne, T., Nieto-Delgado, C. (2017). Adsorptive removal of sulfate from acid mine drainage by polypyrrole modified activated carbons: Effects of polypyrrole deposition protocols and activated carbon source. Chemosphere, 184, 429–437. doi: https://doi.org/10.1016/j.chemosphere.2017.06.019
- Zhu, M., Yin, X., Chen, W., Yi, Z., Tian, H. (2018). Removal of sulphate from mine waters by electrocoagulation/rice straw activated carbon adsorption coupling in a batch system: optimization of process via response surface methodology. Journal of Water Reuse and Desalination, 9 (2), 163–172. doi: https://doi.org/10.2166/wrd.2018.054
- Rahmati, M., Yeganeh, G., Esmaeili, H. (2019). Sulfate Ion Removal From Water Using Activated Carbon Powder Prepared by Ziziphus Spina-Christi Lotus Leaf. Acta Chimica Slovenica, 66 (4), 888–898. doi: https://doi.org/10.17344/acsi.2019.5093
- Wadmare, V. B., Gadhe, K. S., Joshi, M. M. (2019). Studies on physical and chemical composition of Broccoli (Brassica oleracea L.). Int. J. Chem. Stud., 7 (2), 825–828. Available at: https://www.chemijournal.com/archives/2019/vol7issue2/PartO/7-1-654-563.pdf
- Rangel, C. N., Carvalho, L. M. J. de, Fonseca, R. B. F., Soares, A. G., Jesus, E. O. de. (2011). Nutritional value of organic acid lime juice (Citrus latifolia T.), cv. Tahiti. Food Science and Technology (Campinas), 31 (4), 918–922. doi: https://doi.org/10.1590/s0101-20612011000400014
- A review of “Applied Statistics and Probability for Engineers” Douglas C. Montgomery, George C. Runger, 1994 New York, Chichester, John Wiley and Sons ISBN 0 471 01021 9. (1994). European Journal of Engineering Education, 19 (3), 383–383. doi: https://doi.org/10.1080/03043799408928333
- Özbay, N., Yargıç, A. Ş., Yarbay-Şahin, R. Z., Önal, E. (2013). Full Factorial Experimental Design Analysis of Reactive Dye Removal by Carbon Adsorption. Journal of Chemistry, 2013, 1–13. doi: https://doi.org/10.1155/2013/234904
- Koehlert, K. (2017). Activated carbon: Fundamentals and new applications. Chem. Eng. (United States), 124 (7), 32–40.
- Liu, S. X., Chen, X., Chen, X. Y., Liu, Z. F., Wang, H. L. (2007). Activated carbon with excellent chromium(VI) adsorption performance prepared by acid–base surface modification. Journal of Hazardous Materials, 141 (1), 315–319. doi: https://doi.org/10.1016/j.jhazmat.2006.07.006
- Ilomuanya, M., Nashiru, B., Ifudu, N., Igwilo, C. (2017). Effect of pore size and morphology of activated charcoal prepared from midribs of Elaeis guineensis on adsorption of poisons using metronidazole and Escherichia coli O157:H7 as a case study. Journal of Microscopy and Ultrastructure, 5 (1), 32. doi: https://doi.org/10.1016/j.jmau.2016.05.001
- Boehm, H. P. (2002). Surface oxides on carbon and their analysis: a critical assessment. Carbon, 40 (2), 145–149. doi: https://doi.org/10.1016/s0008-6223(01)00165-8
- Shafeeyan, M. S., Daud, W. M. A. W., Houshmand, A., Shamiri, A. (2010). A review on surface modification of activated carbon for carbon dioxide adsorption. Journal of Analytical and Applied Pyrolysis, 89 (2), 143–151. doi: https://doi.org/10.1016/j.jaap.2010.07.006
- Montes-Morán, M. A., Suárez, D., Menéndez, J. A., Fuente, E. (2004). On the nature of basic sites on carbon surfaces: an overview. Carbon, 42 (7), 1219–1225. doi: https://doi.org/10.1016/j.carbon.2004.01.023
- Johnson, J. S., Westmoreland, C. G., Sweeton, F. H., Kraus, K. A., Hagaman, E. W., Eatherly, W. P., Child, H. R. (1986). Modification of cation-exchange properties of activated carbon by treatment with nitric acid. Journal of Chromatography A, 354, 231–248. doi: https://doi.org/10.1016/s0021-9673(01)87025-4
- Leon y Leon, C. A., Solar, J. M., Calemma, V., Radovic, L. R. (1992). Evidence for the protonation of basal plane sites on carbon. Carbon, 30 (5), 797–811. doi: https://doi.org/10.1016/0008-6223(92)90164-r
- Arrigo, R., Hävecker, M., Wrabetz, S., Blume, R., Lerch, M., McGregor, J. et al. (2010). Tuning the Acid/Base Properties of Nanocarbons by Functionalization via Amination. Journal of the American Chemical Society, 132 (28), 9616–9630. doi: https://doi.org/10.1021/ja910169v
- Fujimura, Y., Iino, M. (2008). The surface tension of water under high magnetic fields. Journal of Applied Physics, 103 (12), 124903. doi: https://doi.org/10.1063/1.2940128
- Patel, C. R. P., Tripathi, P., Vishwakarma, A. K., Talat, M., Soni, P. K., Yadav, T. P., Srivastava, O. N. (2018). Enhanced hydrogen generation by water electrolysis employing carbon nano-structure composites. International Journal of Hydrogen Energy, 43 (6), 3180–3189. doi: https://doi.org/10.1016/j.ijhydene.2017.12.142
- Alfarra, A., Frackowiak, E., Béguin, F. (2004). The HSAB concept as a means to interpret the adsorption of metal ions onto activated carbons. Applied Surface Science, 228 (1-4), 84–92. doi: https://doi.org/10.1016/j.apsusc.2003.12.033
- Yakout, S. M., Salem, N. A., Mostafa, A. A., Abdeltawab, A. A. (2018). Relation between biochar physicochemical characteristics on the adsorption of fluoride, nitrite, and nitrate anions from aqueous solution. Particulate Science and Technology, 37 (1), 118–122. doi: https://doi.org/10.1080/02726351.2017.1352633
- Purnami, Hamidi, N., Sasongko, M. N., Widhiyanuriyawan, D., Wardana, I. N. G. (2020). Strengthening external magnetic fields with activated carbon graphene for increasing hydrogen production in water electrolysis. International Journal of Hydrogen Energy, 45 (38), 19370–19380. doi: https://doi.org/10.1016/j.ijhydene.2020.05.148
- Nieto-Delgado, C., Rangel-Mendez, J. R. (2011). Production of activated carbon from organic by-products from the alcoholic beverage industry: Surface area and hardness optimization by using the response surface methodology. Industrial Crops and Products, 34 (3), 1528–1537. doi: https://doi.org/10.1016/j.indcrop.2011.05.014
- Piai, L., Dykstra, J. E., Adishakti, M. G., Blokland, M., Langenhoff, A. A. M., van der Wal, A. (2019). Diffusion of hydrophilic organic micropollutants in granular activated carbon with different pore sizes. Water Research, 162, 518–527. doi: https://doi.org/10.1016/j.watres.2019.06.012
- Satrio, N. W., Winarto, Sugiono, Wardana, I. N. G. (2020). The role of turmeric and bicnat on hydrogen production in porous tofu waste suspension electrolysis. Biomass Conversion and Biorefinery, 12 (7), 2417–2429. doi: https://doi.org/10.1007/s13399-020-00803-0
- Li, L., Quinlivan, P. A., Knappe, D. R. U. (2002). Effects of activated carbon surface chemistry and pore structure on the adsorption of organic contaminants from aqueous solution. Carbon, 40 (12), 2085–2100. doi: https://doi.org/10.1016/s0008-6223(02)00069-6
- Dougherty, D. A. (2012). The Cation−π Interaction. Accounts of Chemical Research, 46 (4), 885–893. doi: https://doi.org/10.1021/ar300265y
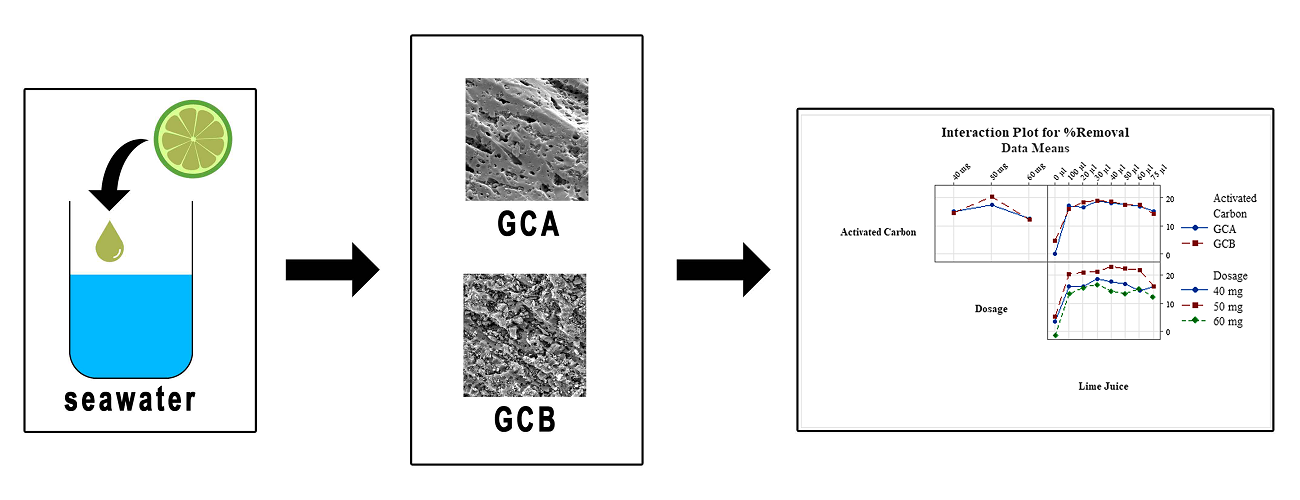
Downloads
Published
How to Cite
Issue
Section
License
Copyright (c) 2023 Yurida Ekawati, Oyong Novareza, Putu Hadi Setyarini, Willy Satrio Nugroho, I Nyoman Gede Wardana

This work is licensed under a Creative Commons Attribution 4.0 International License.
The consolidation and conditions for the transfer of copyright (identification of authorship) is carried out in the License Agreement. In particular, the authors reserve the right to the authorship of their manuscript and transfer the first publication of this work to the journal under the terms of the Creative Commons CC BY license. At the same time, they have the right to conclude on their own additional agreements concerning the non-exclusive distribution of the work in the form in which it was published by this journal, but provided that the link to the first publication of the article in this journal is preserved.
A license agreement is a document in which the author warrants that he/she owns all copyright for the work (manuscript, article, etc.).
The authors, signing the License Agreement with TECHNOLOGY CENTER PC, have all rights to the further use of their work, provided that they link to our edition in which the work was published.
According to the terms of the License Agreement, the Publisher TECHNOLOGY CENTER PC does not take away your copyrights and receives permission from the authors to use and dissemination of the publication through the world's scientific resources (own electronic resources, scientometric databases, repositories, libraries, etc.).
In the absence of a signed License Agreement or in the absence of this agreement of identifiers allowing to identify the identity of the author, the editors have no right to work with the manuscript.
It is important to remember that there is another type of agreement between authors and publishers – when copyright is transferred from the authors to the publisher. In this case, the authors lose ownership of their work and may not use it in any way.