Construction of the stochastic model of thermal radiation from a flammable liquid spill fire
DOI:
https://doi.org/10.15587/1729-4061.2023.288341Keywords:
flammable liquid spill, spill fire, stochastic model, heat flowAbstract
The object of this study is the process of liquid combustion in the pool, and the subject of the study is the characteristics of the random process that describes the heat flow by radiation. Such, in particular, are the law of distribution, mathematical expectation, and correlation function. An experimental study of the combustion of used motor oil in a pool measuring 9.5×8.7 m2 was carried out. The mathematical expectation and variance of the cross-sectional area of the flame were determined by video recording followed by the analysis of individual frames. Testing of the hypothesis about the normal law of distribution of the cross-sectional area showed that with a confidence probability of 0.95, the proposed hypothesis does not contradict the experimental data. A selective correlation function and its approximation in the form of σ2exp(–α|τ|) were constructed. Due to the linear relationship between the cross-sectional area and the heat flux by radiation from the fire, the latter will also have a normal distribution law. At the same time, the magnitude of pulsations (the ratio of the rms deviation to the mathematical expectation) for these random processes will be the same. The value of the parameter α of the correlation function will also be the same.
Taking into account the inertial properties of the device for measuring the heat flux density, a comparison of the experimentally measured values of the heat flux density with the calculated ones was carried out. The measurement results fall into the intervals corresponding to the confidence probability of 0.95. At the same time, the maximum deviation between calculated and experimental data is 14 %. From a practical point of view, the built stochastic model opens up possibilities for taking into account random flame pulsations when determining safe zones for the location of personnel and equipment. The model can be used to specify the thermal effect of fire on steel and concrete structures
References
- Raja, S., Tauseef, S. M., Abbasi, T., Abbasi, S. A. (2018). Risk of Fuel Spills and the Transient Models of Spill Area Forecasting. Journal of Failure Analysis and Prevention, 18 (2), 445–455. doi: https://doi.org/10.1007/s11668-018-0429-1
- Migalenko, K., Nuianzin, V., Zemlianskyi, A., Dominik, A., Pozdieiev, S. (2018). Development of the technique for restricting the propagation of fire in natural peat ecosystems. Eastern-European Journal of Enterprise Technologies, 1 (10 (91)), 31–37. doi: https://doi.org/10.15587/1729-4061.2018.121727
- Vasilchenko, A., Otrosh, Y., Adamenko, N., Doronin, E., Kovalov, A. (2018). Feature of fire resistance calculation of steel structures with intumescent coating. MATEC Web of Conferences, 230, 02036. doi: https://doi.org/10.1051/matecconf/201823002036
- Loboichenko, V., Strelec, V. (2018). The natural waters and aqueous solutions express-identification as element of determination of possible emergency situation. Water and Energy International, 61 (9), 43–50. Available at: http://repositsc.nuczu.edu.ua/handle/123456789/10156
- Kustov, M. V., Kalugin, V. D., Tutunik, V. V., Tarakhno, E. V. (2019). Physicochemical principles of the technology of modified pyrotechnic compositions to reduce the chemical pollution of the atmosphere. Voprosy Khimii i Khimicheskoi Tekhnologii, 1, 92–99. doi: https://doi.org/10.32434/0321-4095-2019-122-1-92-99
- Popov, O., Iatsyshyn, A., Kovach, V., Artemchuk, V., Kameneva, I., Taraduda, D. et al. (2020). Risk Assessment for the Population of Kyiv, Ukraine as a Result of Atmospheric Air Pollution. Journal of Health and Pollution, 10 (25), 200303. doi: https://doi.org/10.5696/2156-9614-10.25.200303
- Liu, J., Li, D., Wang, Z., Chai, X. (2021). A state-of-the-art research progress and prospect of liquid fuel spill fires. Case Studies in Thermal Engineering, 28, 101421. doi: https://doi.org/10.1016/j.csite.2021.101421
- Chen, Y., Fang, J., Zhang, X., Miao, Y., Lin, Y., Tu, R., Hu, L. (2023). Pool fire dynamics: Principles, models and recent advances. Progress in Energy and Combustion Science, 95, 101070. doi: https://doi.org/10.1016/j.pecs.2022.101070
- Guo, Y., Xiao, G., Wang, L., Chen, C., Deng, H., Mi, H. et al. (2023). Pool fire burning characteristics and risks under wind-free conditions: State-of-the-art. Fire Safety Journal, 136, 103755. doi: https://doi.org/10.1016/j.firesaf.2023.103755
- Huang, X., Huang, T., Zhuo, X., Tang, F., He, L., Wen, J. (2021). A global model for flame pulsation frequency of buoyancy-controlled rectangular gas fuel fire with different boundaries. Fuel, 289, 119857. doi: https://doi.org/10.1016/j.fuel.2020.119857
- Zhang, X., Fang, X., Miao, Y., Hu, L. (2020). Experimental study on pulsation frequency of free-, wall- and corner buoyant turbulent diffusion flames. Fuel, 276, 118022. doi: https://doi.org/10.1016/j.fuel.2020.118022
- Biswas, K., Zheng, Y., Kim, C. H., Gore, J. (2007). Stochastic time series analysis of pulsating buoyant pool fires. Proceedings of the Combustion Institute, 31 (2), 2581–2588. doi: https://doi.org/10.1016/j.proci.2006.07.234
- Sun, X., Hu, L., Zhang, X., Ren, F., Yang, Y., Fang, X. (2021). Experimental study on flame pulsation behavior of external venting facade fire ejected from opening of a compartment. Proceedings of the Combustion Institute, 38 (3), 4485–4493. doi: https://doi.org/10.1016/j.proci.2020.06.181
- Kovalov, A., Otrosh, Y., Rybka, E., Kovalevska, T., Togobytska, V., Rolin, I. (2020). Treatment of Determination Method for Strength Characteristics of Reinforcing Steel by Using Thread Cutting Method after Temperature Influence. Materials Science Forum, 1006, 179–184. doi: https://doi.org/10.4028/www.scientific.net/msf.1006.179
- Abramov, Y. A., Basmanov, O. E., Salamov, J., Mikhayluk, A. A. (2018). Model of thermal effect of fire within a dike on the oil tank. Naukovyi Visnyk Natsionalnoho Hirnychoho Universytetu, 2, 95–101. doi: https://doi.org/10.29202/nvngu/2018-2/12
- Abramov, Y., Basmanov, O., Salamov, J., Mikhayluk, A., Yashchenko, O. (2019). Developing a model of tank cooling by water jets from hydraulic monitors under conditions of fire. Eastern-European Journal of Enterprise Technologies, 1 (10 (97)), 14–20. doi: https://doi.org/10.15587/1729-4061.2019.154669
- Abramov, Y., Basmanov, O., Oliinik, V., Khmyrov, I., Khmyrova, A. (2022). Modeling the convective component of the heat flow from a spill fire at railway accidence. EUREKA: Physics and Engineering, 6, 128–138. doi: https://doi.org/10.21303/2461-4262.2022.002702
- Shen, G., Zhou, K., Wu, F., Jiang, J., Dou, Z. (2018). A Model Considering the Flame Volume for Prediction of Thermal Radiation from Pool Fire. Fire Technology, 55 (1), 129–148. doi: https://doi.org/10.1007/s10694-018-0779-y
- Ji, J., Ge, F., Qiu, T. (2021). Experimental and theoretical research on flame emissivity and radiative heat flux from heptane pool fires. Proceedings of the Combustion Institute, 38 (3), 4877–4885. doi: https://doi.org/10.1016/j.proci.2020.05.052
- Li, Y., Jiang, J., Zhang, Q., Yu, Y., Wang, Z., Liu, H., Shu, C.-M. (2019). Static and dynamic flame model effects on thermal buckling: Fixed-roof tanks adjacent to an ethanol pool-fire. Process Safety and Environmental Protection, 127, 23–35. doi: https://doi.org/10.1016/j.psep.2019.05.001
- Abramov, Y., Basmanov, O., Oliinik, V., Kolokolov, V. (2022). Stochastic model of heating the shell of a tank under the thermal effect of a fire. Problems of Emergency Situations, 35, 4–16. doi: https://doi.org/10.52363/2524-0226-2022-35-1
- Abramov, Yu. A., Basmanov, A. E. (2006). Otsenka pul'satsiy plameni pri gorenii nefteproduktov. Radioelektronika i informatika, 1 (32), 40–42. Available at: http://repositsc.nuczu.edu.ua/handle/123456789/278
- Drysdale, D. (2011). An Introduction to Fire Dynamics. John Wiley & Sons. doi: https://doi.org/10.1002/9781119975465
- Otrosh, Y., Semkiv, O., Rybka, E., Kovalov, A. (2019). About need of calculations for the steel framework building in temperature influences conditions. IOP Conference Series: Materials Science and Engineering, 708 (1), 012065. doi: https://doi.org/10.1088/1757-899x/708/1/012065
- Pospelov, B., Andronov, V., Rybka, E., Skliarov, S. (2017). Design of fire detectors capable of self-adjusting by ignition. Eastern-European Journal of Enterprise Technologies, 4 (9 (88)), 53–59. doi: https://doi.org/10.15587/1729-4061.2017.108448
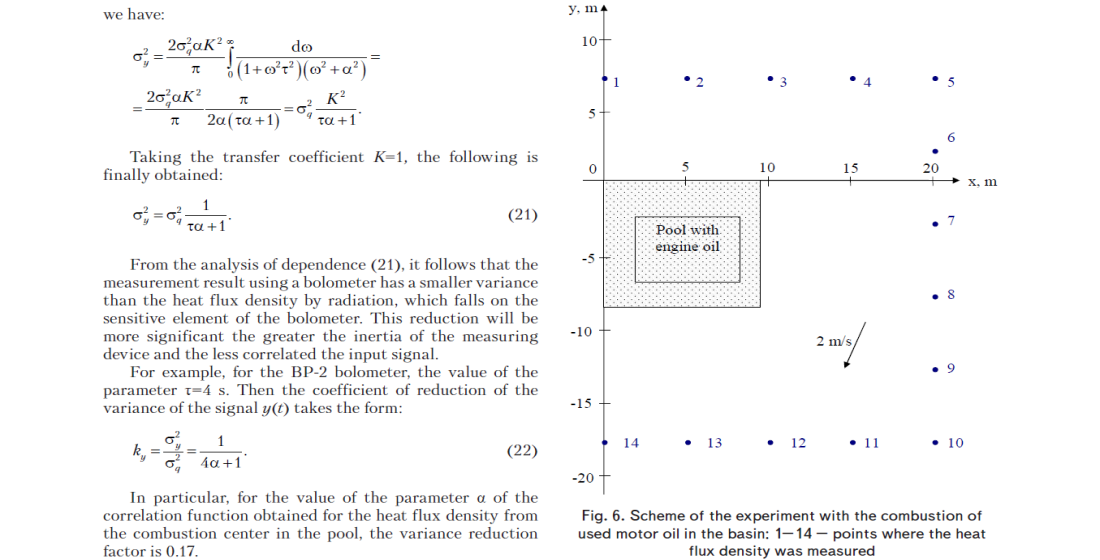
Downloads
Published
How to Cite
Issue
Section
License
Copyright (c) 2023 Volodymyr Oliinyk

This work is licensed under a Creative Commons Attribution 4.0 International License.
The consolidation and conditions for the transfer of copyright (identification of authorship) is carried out in the License Agreement. In particular, the authors reserve the right to the authorship of their manuscript and transfer the first publication of this work to the journal under the terms of the Creative Commons CC BY license. At the same time, they have the right to conclude on their own additional agreements concerning the non-exclusive distribution of the work in the form in which it was published by this journal, but provided that the link to the first publication of the article in this journal is preserved.
A license agreement is a document in which the author warrants that he/she owns all copyright for the work (manuscript, article, etc.).
The authors, signing the License Agreement with TECHNOLOGY CENTER PC, have all rights to the further use of their work, provided that they link to our edition in which the work was published.
According to the terms of the License Agreement, the Publisher TECHNOLOGY CENTER PC does not take away your copyrights and receives permission from the authors to use and dissemination of the publication through the world's scientific resources (own electronic resources, scientometric databases, repositories, libraries, etc.).
In the absence of a signed License Agreement or in the absence of this agreement of identifiers allowing to identify the identity of the author, the editors have no right to work with the manuscript.
It is important to remember that there is another type of agreement between authors and publishers – when copyright is transferred from the authors to the publisher. In this case, the authors lose ownership of their work and may not use it in any way.