Expansion of the functional capacities of electrostatic mirror analyzers for electron spectroscopy
DOI:
https://doi.org/10.15587/1729-4061.2023.289781Keywords:
electron spectroscopy, spherical and cylindrical mirrors, energy analyzerAbstract
Electron spectroscopy methods are widely used in scientific research and for technological purposes. The main element of spectrometers is an analyzer of charged particle beams. Electrostatic mirror systems are widely used due to their simpler practical realization. At their development two purposes were set: to improve the quality of spatial focusing of charged particles or to increase the value of linear energy dispersion. The objects of the study are electrostatic systems characterized by small sizes, simplicity of stabilization and localization of the working field and its shielding from the external electromagnetic disturbances. From all the known types of energy analyzers, suitable for the analysis of solid surfaces, preference is given to those that have good electron-optical properties, are simple in manufacture and operation. Therefore, spherical and cylindrical mirrors, which have become a basic tool for firms producing spectrometers, have been chosen as the object of study. The work solves the problem of expansion of the functional capacities of these systems by, firstly, combining several research methods in one device; and secondly, by solving specific narrower problems. A photoelectron or Auger spectrometer with an increased scanning area is proposed, where the initial angular opening of the beam 4° after passing a cylindrical mirror is increased to 10°, and the image smearing is reduced to 0.05 %. An Auger-electron spectrometer for analysis of rough surface has been developed, which allows to increase the probing depths by more than 5 times. A double filter type energy analyzer is calculated. Energy resolution was improved to 1.37 % by eliminating potential barrier smearing in low energy filter mode. Previously, the energy resolution was limited to 10 % due to this drawback
References
- Greczynski, G., Hultman, L. (2020). X-ray photoelectron spectroscopy: Towards reliable binding energy referencing. Progress in Materials Science, 107, 100591. doi: https://doi.org/10.1016/j.pmatsci.2019.100591
- Fadley, C. S. (2009). X-ray photoelectron spectroscopy: From origins to future directions. Nuclear Instruments and Methods in Physics Research Section A: Accelerators, Spectrometers, Detectors and Associated Equipment, 601 (1-2), 8–31. doi:https://doi.org/10.1016/j.nima.2008.12.189
- Bolli, E., Kaciulis, S., Mezzi, A. (2020). ESCA as a Tool for Exploration of Metals’ Surface. Coatings, 10 (12), 1182. doi: https://doi.org/10.3390/coatings10121182
- Scheithauer, U. (2020). Experimental setups for XPS measurements beyond the instrumental lateral resolution limit. Surface and Interface Analysis, 52 (12), 1185–1190. doi: https://doi.org/10.1002/sia.6828
- Diehl, R. D., Ledieu, J., Ferralis, N., Szmodis, A. W., McGrath, R. (2003). Low-energy electron diffraction from quasicrystal surfaces. Journal of Physics: Condensed Matter, 15 (3), R63–R81. doi: https://doi.org/10.1088/0953-8984/15/3/201
- Schweizer, P., Denninger, P., Dolle, C., Spiecker, E. (2020). Low energy nano diffraction (LEND) – A versatile diffraction technique in SEM. Ultramicroscopy, 213, 112956. doi: https://doi.org/10.1016/j.ultramic.2020.112956
- Powell, C. J. (2003). Growth and trends in Auger-electron spectroscopy and x-ray photoelectron spectroscopy for surface analysis. Journal of Vacuum Science & Technology A: Vacuum, Surfaces, and Films, 21 (5), S42–S53. doi: https://doi.org/10.1116/1.1599862
- Unger, W. E. S., Wirth, T., Hodoroaba, V.-D. (2020). Auger electron spectroscopy. Characterization of Nanoparticles, 373–395. doi: https://doi.org/10.1016/b978-0-12-814182-3.00020-1
- Fletcher, J. S., Vickerman, J. C. (2012). Secondary Ion Mass Spectrometry: Characterizing Complex Samples in Two and Three Dimensions. Analytical Chemistry, 85 (2), 610–639. doi: https://doi.org/10.1021/ac303088m
- Brais, C. J., Ibañez, J. O., Schwartz, A. J., Ray, S. J. (2020). Recent advances in instrumental approaches to time‐of‐flight mass spectrometry. Mass Spectrometry Reviews, 40 (5), 647–669. doi: https://doi.org/10.1002/mas.21650
- Gurov, V. S., Saulebekov, A. O., Trubitsyn, A. A. (2015). Analytical, Approximate-Analytical, and Numerical Methods for Design of Energy Analyzers. Advances in Imaging and Electron Physics. doi: https://doi.org/10.1016/s1076-5670(15)00103-2
- Gorelik, V. (2023). Optimization of cylindrical mirror analyzer. Journal of Electron Spectroscopy and Related Phenomena, 264, 147315. doi: https://doi.org/10.1016/j.elspec.2023.147315
- Edwards, D. (2016). The segmented cylindrical mirror analyzer (CMA). Journal of Electron Spectroscopy and Related Phenomena, 209, 46–52. doi: https://doi.org/10.1016/j.elspec.2016.02.004
- Baranova, L. A. (2017). Improved cylindrical mirror energy analyzer. Technical Physics, 62 (3), 480–483. doi: https://doi.org/10.1134/s1063784217030057
- Guseinov, N. R., Ilyin, A. M. (2021). Electrostatic energy analyzer for nanotechnology applications. Journal of Electron Spectroscopy and Related Phenomena, 246, 147031. doi: https://doi.org/10.1016/j.elspec.2020.147031
- Kobayashi, E., Seo, J., Nambu, A., Mase, K. (2007). Development of a miniature double-pass cylindrical mirror electron energy analyzer (DPCMA), and its application to Auger photoelectron coincidence spectroscopy (APECS). Surface Science, 601 (17), 3589–3592. doi: https://doi.org/10.1016/j.susc.2007.06.073
- Davydov, S. N., Danilov, M. M., Korablev, V. V. (1999). Spherical mirror analyzer as an instrument for electron coincidence spectroscopy. Technical Physics, 44 (1), 99–103. doi: https://doi.org/10.1134/1.1259259
- Artamonov, O. M., Samarin, S. N., Paolicelli, G., Stefani, G. (2003). The use of the time–energy dispersion in an electron energy analyzer. Journal of Electron Spectroscopy and Related Phenomena, 131-132, 105–116. doi: https://doi.org/10.1016/s0368-2048(03)00129-4
- Nesvidomin, A., Pylypaka, S., Volina, T., Kalenyk, M., Shuliak, I., Semirnenko, Y. et al. (2023). Constructing geometrical models of spherical analogs of the involute of a circle and cycloid. Eastern-European Journal of Enterprise Technologies, 4 (7 (124)), 6–12. doi: https://doi.org/10.15587/1729-4061.2023.284982
- Saulebekov, А. О. (2019). Development of energy analyzer of charged particles based on the basis non-uniform electrostatic field. Eurasian Physical Technical Journal, 16 (1), 24–29. doi: https://doi.org/10.31489/2019no1/24-29
- Kambarova, Z., Saulebekov, A. (2020). Development of a Mirror Energy Analyzer of Charged Particles Beams Based on a Modified Electrostatic Field. 2020 7th International Congress on Energy Fluxes and Radiation Effects (EFRE). doi: https://doi.org/10.1109/efre47760.2020.9242029
- Kambarova, Z., Saulebekov, A. (2020). Analyzer of Charged Particles Based on the Electrostatic Quadrupole-Cylindrical Field in the «Axis-Ring» Focusing Regime. 2020 7th International Congress on Energy Fluxes and Radiation Effects (EFRE). doi: https://doi.org/10.1109/efre47760.2020.9242088
- Kambarova, Zh. T., Trubitsyn, A. A., Saulebekov, A. O. (2018). Axially Symmetric Energy Analyzer Based on the Electrostatic Decapole-Cylindrical Field. Technical Physics, 63 (11), 1667–1671. doi: https://doi.org/10.1134/s1063784218110142
- Ashimbaeva, B. U., Chokin, K. Sh., Saulebekov, A. O., Kambarova, Zh. T. (2012). The combined energy analyzer composed of electrostatic mirror fields. Journal of Electron Spectroscopy and Related Phenomena, 185 (11), 518–522. doi: https://doi.org/10.1016/j.elspec.2012.10.008
- Ashimbayeva, B. U., Chokin, K. Sh., Saulebekov, A. O., Kambarova, Zh. T. (2012). Modelirovaniye elektrostaticheskoy sistemy iz tsilindricheskogo i giperbolicheskogo zerkal. Prikladnaya fizika (Applied Physics), 4, 73–78.
- Photoelectron spectrometer, ESCALAB Mk II by Vacuum Generators. Available at: https://jacobs.physik.uni-saarland.de/home/index.php?page=steinbeiss/home_cms_steinbeissdet3-1&navi=service
- Getman, A. (2018). Development of the technique for improving the structure of a magnetic field in the aperture of a quadrupole electromagnet with a superconducting winding. Eastern-European Journal of Enterprise Technologies, 5 (5 (95)), 6–12. doi: https://doi.org/10.15587/1729-4061.2018.142163
- Trubitsyn, A. A., Tolstoguzov, A. B., Saulebekov, A. O., Suvorov, D. V., Tarabrin, D. Yu., Kambarova, Zh. T., Kuksa, P. I. (2012). Proyektirovaniye dlinnofokusnogo ozhe-mikrozonda. Vestnik Ryazanskogo gosudarstvennogo radiotekhnicheskogo universiteta, 42 (4 (1)), 54–59.
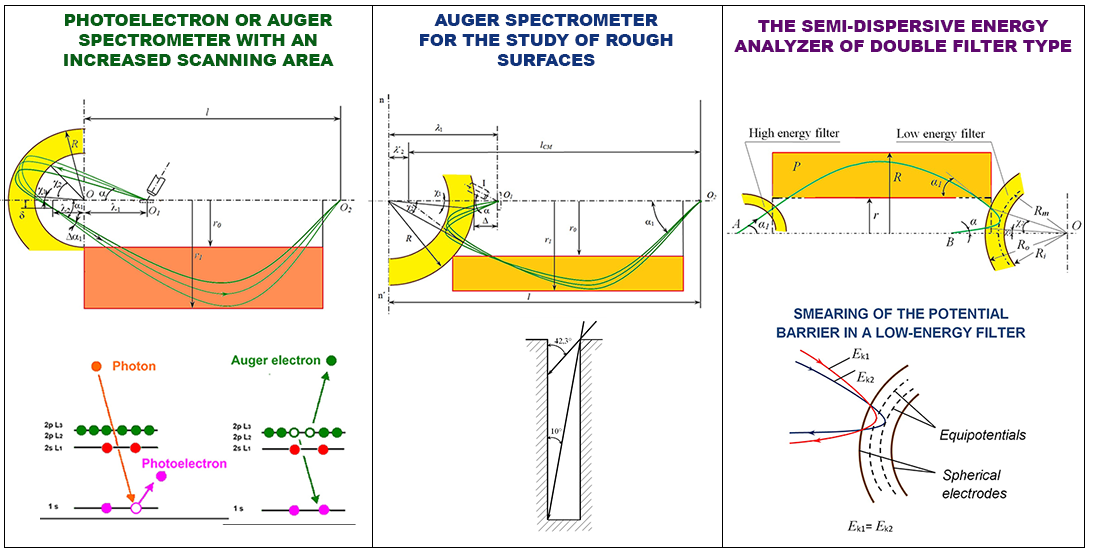
Downloads
Published
How to Cite
Issue
Section
License
Copyright (c) 2023 Zhanar Kambarova

This work is licensed under a Creative Commons Attribution 4.0 International License.
The consolidation and conditions for the transfer of copyright (identification of authorship) is carried out in the License Agreement. In particular, the authors reserve the right to the authorship of their manuscript and transfer the first publication of this work to the journal under the terms of the Creative Commons CC BY license. At the same time, they have the right to conclude on their own additional agreements concerning the non-exclusive distribution of the work in the form in which it was published by this journal, but provided that the link to the first publication of the article in this journal is preserved.
A license agreement is a document in which the author warrants that he/she owns all copyright for the work (manuscript, article, etc.).
The authors, signing the License Agreement with TECHNOLOGY CENTER PC, have all rights to the further use of their work, provided that they link to our edition in which the work was published.
According to the terms of the License Agreement, the Publisher TECHNOLOGY CENTER PC does not take away your copyrights and receives permission from the authors to use and dissemination of the publication through the world's scientific resources (own electronic resources, scientometric databases, repositories, libraries, etc.).
In the absence of a signed License Agreement or in the absence of this agreement of identifiers allowing to identify the identity of the author, the editors have no right to work with the manuscript.
It is important to remember that there is another type of agreement between authors and publishers – when copyright is transferred from the authors to the publisher. In this case, the authors lose ownership of their work and may not use it in any way.