Synthesis of nanocarbon by high-voltage breakdown of hydrocarbons
DOI:
https://doi.org/10.15587/1729-4061.2023.292774Keywords:
synthesis of nanocarbon, high-voltage breakdown of hydrocarbons, mass yield of the product, electromagnetic propertiesAbstract
The object of research is the mechanisms of synthesis of nanocarbon structures in the process of electric high-voltage breakdown of hydrocarbons.
The problem to be solved is the purposeful synthesis of various types of nanocarbon with small losses of raw materials.
Mechanisms of nanocarbon formation in the process of high-voltage electric breakdown of hydrocarbons have been established. It is shown that a high-voltage breakdown leads to a cascade of chemical transformations. As a result of transformations, lower gaseous hydrocarbons are formed due to the destruction of molecules and higher ones – as a result of polymerization, and as a result of dehydrocyclization and polymerization with the participation of metal catalysts – various carbon nanostructures. The possibility of targeted synthesis of fullerene-like structures, nanotubes with diameters from 10 to 50 nm, nanofibers, and films is demonstrated. Experimental studies have confirmed that the qualitative and quantitative composition of nanocarbon can be varied in a wide range. With an increase in the number of carbon atoms or the number of C–C bonds in the raw material molecules, other things being equal, the practical yield of solid nanocarbon increases. It was determined that the synthesis of structured nanocarbon from a mixture of hydrocarbon gases, formed as a result of high-voltage breakdown of liquid hydrocarbons, actively occurs on the nickel-chromium catalytic surface. An increase in the area of the catalytic deposition surface leads to an increase in the yield of nanocarbon. The study of the ability of the obtained nanocarbon samples to absorb electromagnetic radiation confirmed the potential of the method of high-voltage breakdown of hydrocarbons for the synthesis of materials that weaken electromagnetic radiation at a frequency of 25 to 38 GHz. The greatest weakening is observed for samples consisting mainly of carbon nanotubes and nickel nanoparticles
References
- Zhang, Z., Ohta, S., Shiba, S., Niwa, O. (2022). Nanocarbon film electrodes for electro-analysis and electrochemical sensors. Current Opinion in Electrochemistry, 35, 101045. doi: https://doi.org/10.1016/j.coelec.2022.101045
- Han, L., Song, Q., Li, K., Yin, X., Sun, J., Li, H. et al. (2021). Hierarchical, seamless, edge-rich nanocarbon hybrid foams for highly efficient electromagnetic-interference shielding. Journal of Materials Science & Technology, 72, 154–161. doi: https://doi.org/10.1016/j.jmst.2020.07.020
- Xiao, Z., Kong, L. B., Ruan, S., Li, X., Yu, S., Li, X. et al. (2018). Recent development in nanocarbon materials for gas sensor applications. Sensors and Actuators B: Chemical, 274, 235–267. doi: https://doi.org/10.1016/j.snb.2018.07.040
- Pašti, I. A., Janošević Ležaić, A., Gavrilov, N. M., Ćirić-Marjanović, G., Mentus, S. V. (2018). Nanocarbons derived from polymers for electrochemical energy conversion and storage – A review. Synthetic Metals, 246, 267–281. doi: https://doi.org/10.1016/j.synthmet.2018.11.003
- Zhang, F., Zhang, H. (2021). Applications of nanocarbons in redox flow batteries. New Carbon Materials, 36 (1), 82–92. doi: https://doi.org/10.1016/s1872-5805(21)60006-9
- Madhu, R., Periasamy, A. P., Schlee, P., Hérou, S., Titirici, M.-M. (2023). Lignin: A sustainable precursor for nanostructured carbon materials for supercapacitors. Carbon, 207, 172–197. doi: https://doi.org/10.1016/j.carbon.2023.03.001
- Liang, Y. N., Oh, W.-D., Li, Y., Hu, X. (2018). Nanocarbons as platforms for developing novel catalytic composites: overview and prospects. Applied Catalysis A: General, 562, 94–105. doi: https://doi.org/10.1016/j.apcata.2018.05.021
- Tang, C., Titirici, M.-M., Zhang, Q. (2017). A review of nanocarbons in energy electrocatalysis: Multifunctional substrates and highly active sites. Journal of Energy Chemistry, 26 (6), 1077–1093. doi: https://doi.org/10.1016/j.jechem.2017.08.008
- Driscoll, N., Dong, R., Vitale, F. (2021). Emerging approaches for sensing and modulating neural activity enabled by nanocarbons and carbides. Current Opinion in Biotechnology, 72, 76–85. doi: https://doi.org/10.1016/j.copbio.2021.10.007
- Yang, N., Jiang, X. (2017). Nanocarbons for DNA sequencing: A review. Carbon, 115, 293–311. doi: https://doi.org/10.1016/j.carbon.2017.01.012
- Elkodous, M. A., Olojede, S. O., Sahoo, S., Kumar, R. (2023). Recent advances in modification of novel carbon-based composites: Synthesis, properties, and biotechnological/ biomedical applications. Chemico-Biological Interactions, 379, 110517. doi: https://doi.org/10.1016/j.cbi.2023.110517
- Zhang, X., Zhao, N., He, C. (2020). The superior mechanical and physical properties of nanocarbon reinforced bulk composites achieved by architecture design – A review. Progress in Materials Science, 113, 100672. doi: https://doi.org/10.1016/j.pmatsci.2020.100672
- Rud, A. D., Kornienko, N. E., Kirian, I. M., Kirichenko, A. N., Kucherov, Oleksandr. P. (2018). Local heteroallotropic structures of carbon. Materials Today: Proceedings, 5 (12), 26089–26095. doi: https://doi.org/10.1016/j.matpr.2018.08.035
- Rud, A. D., Kornienko, N. E., Polunkin, I. V., Boguslavskii, L. Z., Vinnichenko, D. V., Kirian, I. M. et al. (2023). Structure of carbon nanospheres modified with oxygen-containing groups and halogens. Applied Nanoscience, 13 (10), 6929–6937. doi: https://doi.org/10.1007/s13204-023-02817-2
- García-Ruiz, D. L., Granados-Martínez, F. G., Gutiérrez-García, C. J., Ambriz-Torres, J. M., Contreras-Navarrete, J. de J., Flores-Ramírez, N. et al. (2021). “Synthesis of carbon nanomaterials by chemical vapor deposition method using green chemistry principles.” Handbook of Greener Synthesis of Nanomaterials and Compounds, 273–314. doi: https://doi.org/10.1016/b978-0-12-821938-6.00008-6
- Gerberich, W. W., Jungk, J. M., Mook, W. M. (2003). The bottom-up approach to materials by design. Nano and Microstructural Design of Advanced Materials, 211–220. doi: https://doi.org/10.1016/b978-008044373-7/50046-2
- Kumar, R., Pérez del Pino, A., Sahoo, S., Singh, R. K., Tan, W. K., Kar, K. K. et al. (2022). Laser processing of graphene and related materials for energy storage: State of the art and future prospects. Progress in Energy and Combustion Science, 91, 100981. doi: https://doi.org/10.1016/j.pecs.2021.100981
- Wang, P., Hao, X., Tang, B., Abudula, A., Guan, G. (2022). Nanocarbon-based metal-free catalysts. Carbon-Based Metal Free Catalysts, 1–19. doi: https://doi.org/10.1016/b978-0-323-88515-7.00006-7
- Pandey, A., Chauhan, P. (2023). Functionalized graphene nanomaterials: Next-generation nanomedicine. Functionalized Carbon Nanomaterials for Theranostic Applications, 3–18. doi: https://doi.org/10.1016/b978-0-12-824366-4.00020-0
- Woldu, A. R., Huang, Z., Zhao, P., Hu, L., Astruc, D. (2022). Electrochemical CO2 reduction (CO2RR) to multi-carbon products over copper-based catalysts. Coordination Chemistry Reviews, 454, 214340. doi: https://doi.org/10.1016/j.ccr.2021.214340
- Yan, G., Fu, H., Zhao, Y., Sun, Z., Zhang, B. (2022). A review on optimizing potentials of high voltage pulse breakage technology based on electrical breakdown in water. Powder Technology, 404, 117293. doi: https://doi.org/10.1016/j.powtec.2022.117293
- Zhu, L., He, Z.-H., Gao, Z.-W., Tan, F.-L., Yue, X.-G., Chang, J.-S. (2014). Research on the influence of conductivity to pulsed arc electrohydraulic discharge in water. Journal of Electrostatics, 72 (1), 53–58. doi: https://doi.org/10.1016/j.elstat.2013.11.004
- Park, H.-K., Park, D.-H., Chung, B.-J. (2023). Influence of the electrolyte conductivity on the critical current density and the breakdown voltage. Chinese Journal of Chemical Engineering, 59, 169–175. doi: https://doi.org/10.1016/j.cjche.2023.01.007
- Kuskova, N. I., Yushchishina, A. N., Malyushevskaya, A. P., Tsolin, P. L., Petrichenko, L. A., Smal’ko, A. A. (2010). Production of carbonic nanomaterials in the course of electrodischarge treatment of organic liquids. Surface Engineering and Applied Electrochemistry, 46 (2), 149–153. doi: https://doi.org/10.3103/s1068375510020110
- Kuskova, N. I., Baklar’, V. Yu., Terekhov, A. Yu., Yushchishina, A. N., Petrichenko, S. V., Tsolin, P. L., Malyushevskaya, A. P. (2014). Synthesis of carbon nanomaterials from gases generated in the course of the electrodischarge treatment of organic liquids. Surface Engineering and Applied Electrochemistry, 50 (2), 101–105. doi: https://doi.org/10.3103/s1068375514020094
- Kuskova, N. I., Dubovenko, K. V., Petrichenko, S. V., Tsolin, P. L., Chaban, S. O. (2013). Electrodischarge technology and equipment to produce new carbon nanomaterials. Surface Engineering and Applied Electrochemistry, 49 (3), 215–221. doi: https://doi.org/10.3103/s1068375513030095
- Zhao, D., Li, X., Shen, Z. (2008). Microwave absorbing property and complex permittivity and permeability of epoxy composites containing Ni-coated and Ag filled carbon nanotubes. Composites Science and Technology, 68 (14), 2902–2908. doi: https://doi.org/10.1016/j.compscitech.2007.10.006
- Kuskova, N. I., Syzonenko, O. M., Torpakov, A. S. (2020). Electric discharge method of synthesis of carbon and metal–carbon nanomaterials. High Temperature Materials and Processes, 39 (1), 357–367. doi: https://doi.org/10.1515/htmp-2020-0078
- Gaffney, J. S., Marley, N. A. (2018). Kinetics and the Rate of Chemical Reactions. General Chemistry for Engineers, 283–317. doi: https://doi.org/10.1016/b978-0-12-810425-5.00009-6
- Kucherov, O., Rud, A., Gubanov, V., Biliy, M. (2020). Spatial 3d Direct Visualization of Atoms, Molecules and Chemical Bonds. American Journal of Applied Chemistry, 8 (4), 94. doi: https://doi.org/10.11648/j.ajac.20200804.11
- Sergiienko, R., Shibata, E., Akase, Z., Suwa, H., Nakamura, T., Shindo, D. (2006). Carbon encapsulated iron carbide nanoparticles synthesized in ethanol by an electric plasma discharge in an ultrasonic cavitation field. Materials Chemistry and Physics, 98 (1), 34–38. doi: https://doi.org/10.1016/j.matchemphys.2005.08.064
- Dolmatov, V. Yu., Myullyumyaki, V., Vehanen, A. (2013). Vozmozhniy mehanizm obrazovaniya nanoalmaza pri detonatsionnom sinteze. Sverhtverdye materialy, 3, 19–28. Available at: http://dspace.nbuv.gov.ua/handle/123456789/126042
- Shvartsev, S. L. (2009). Self-organizing Abiogenic Dissipative Structures in the Geologic History of the Earth. Earth Science Frontiers, 16 (6), 257–275. doi: https://doi.org/10.1016/s1872-5791(08)60114-1
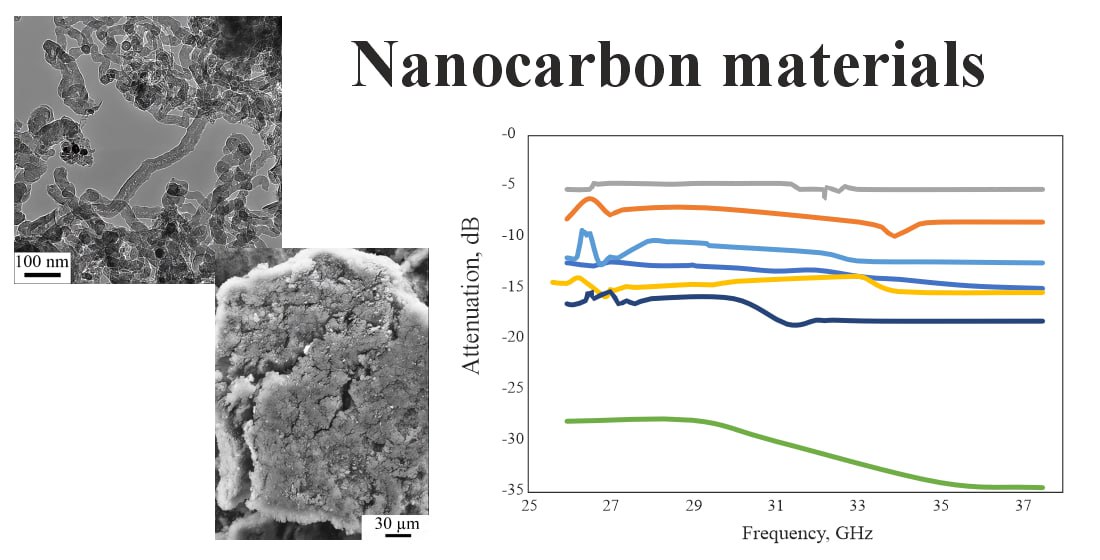
Downloads
Published
How to Cite
Issue
Section
License
Copyright (c) 2023 Nataliya Kuskova, Antonina Malyushevskaya, Mykola Prystash, Svitlana Prystash, Yury Adamchuk

This work is licensed under a Creative Commons Attribution 4.0 International License.
The consolidation and conditions for the transfer of copyright (identification of authorship) is carried out in the License Agreement. In particular, the authors reserve the right to the authorship of their manuscript and transfer the first publication of this work to the journal under the terms of the Creative Commons CC BY license. At the same time, they have the right to conclude on their own additional agreements concerning the non-exclusive distribution of the work in the form in which it was published by this journal, but provided that the link to the first publication of the article in this journal is preserved.
A license agreement is a document in which the author warrants that he/she owns all copyright for the work (manuscript, article, etc.).
The authors, signing the License Agreement with TECHNOLOGY CENTER PC, have all rights to the further use of their work, provided that they link to our edition in which the work was published.
According to the terms of the License Agreement, the Publisher TECHNOLOGY CENTER PC does not take away your copyrights and receives permission from the authors to use and dissemination of the publication through the world's scientific resources (own electronic resources, scientometric databases, repositories, libraries, etc.).
In the absence of a signed License Agreement or in the absence of this agreement of identifiers allowing to identify the identity of the author, the editors have no right to work with the manuscript.
It is important to remember that there is another type of agreement between authors and publishers – when copyright is transferred from the authors to the publisher. In this case, the authors lose ownership of their work and may not use it in any way.