Devising a technique for descending the illumination elements of aerial vehicles
DOI:
https://doi.org/10.15587/1729-4061.2024.303639Keywords:
drag force, dual-rotor impeller, descent speed, illumination time, light intensity, illumination radiusAbstract
The object of this study is the process of descending illumination elements equipped with a braking device in the form of two-bladed grills rotating in different directions. The classic parachute method does not provide the necessary speed of descent, it has low illumination parameters and significant drift of illumination elements by side wind.
To solve the tasks set, mathematical dependences were obtained for calculating the aerodynamic characteristics of the descent device with the illumination element and its delivery to the ejection point. The drag and lift force coefficients during the flow around the blades of a dual-rotor impeller with different Reynolds numbers were determined by the method of numerical modeling based on the ANSYS CFX software package. The optimal geometric characteristics of the profile satisfying the condition for the necessary speed of descent of the illumination element at the given weight of the descent apparatus were determined.
Reasonable requirements for illumination parameters and an improved composition of the flare have been proposed.
A mathematical model of the movement of a body of variable mass to the point of ejection of the illumination element was built.
The new design of the descent device makes it possible to reduce the speed of descent by 10–15 % and increase the weight of the payload by 20–30 %. The proposed illumination composition provides sufficient illumination of the object for 5 minutes with a light intensity of 2–2,5 million candelas and an average diameter of the illuminated area of 2000–2500 m. The mathematical model of the movement of a variable mass body to the point of the illumination element ejection makes it possible to determine with high accuracy the gun firing settings with illumination ammunition (30–40 % more accurate) and the time of ejection of illumination elements.
Results of the current research make it possible to solve the scientific problem of ensuring the maximum efficiency of illuminating the terrain at night
References
- Tkachuk, P. P., Chumakevych, V. O., Droban, O. M., Fedor, B. S., Yevdokimov, P. M. (2023). Boieprypasy. Kyiv: Vyd. dim «SKIF», 266. Available at: https://jurkniga.ua/contents/boiepripasi-pidruchnik.pdf
- Buhaieva, L. M., Boiko, T. V., Beznosyk, Yu. O. (2017). Systemnyi analiz khimiko-tekhnolohichnykh kompleksiv. Kyiv: Interservis, 254. Available at: https://www.sworld.com.ua/doi/sahtk.pdf
- Dmitrievskiy, A. A. (2005). Vneshnyaya ballistika. Moscow: Mashinostroenie, 607.
- Homylev, S. A., Reznik, S. B., Ershov, S. V. (2008). Chislennoe issledovanie obtekaniya turbinnyh reshetok profiley: chast' 2 – issledovanie harakteristik vysokonagruzhennyh reshetok. Aviatsionno-kosmicheskaya tehnika i tehnologiya, 8 (55), 46–50. Available at: http://nbuv.gov.ua/UJRN/aktit_2008_8_12
- Makeiev, V. I., Raskoshnyi, A. F. (2023). Optymizatsiya konstruktyvnykh parametriv osvitliuvalnykh litalnykh aparativ dlia zmenshennia yikh rozsiiuvannia. Zbirnyk naukovykh prats TsNDI OVT ZSU, 1 (88), 83–92.
- Tiahniy, V. H., Yemets, V. V. (2023). Osnovy aerodynamiky ta dynamiky polotu: ch. I: Aerohidrohazodynamika. Kharkiv: KhNUVS, 280. Available at: https://dspace.univd.edu.ua/items/c3726f93-7e24-4fb0-8c62-e93f90aace8b
- Makeiev, V. I., Kolobylin, S. M., Zhytnyk, V. Ye., Liapa, M. M. (2023). Pat. No. 153331 UA. Artyleriiskyi snariad. No. u202201954; declareted: 09.06.2022; published: 21.06.2023, Bul. No. 25/2023. Available at: https://base.uipv.org/searchINV/search.php?action=viewdetails&IdClaim=285443
- Rusanov, A. V., Kos'yanova, A. I., Suhorebryy, P. N., Horev, O. N. (2013). Gazodinamicheskoe sovershenstvovanie protochnoy chasti tsilindra vysokogo davleniya parovoy turbiny K-325-23,5. Nauka ta innovatsiyi, 9 (1), 33–40.
- Navin Kumar, B., Parammasivam, K. M., Selvaraju, P. N., Anbalagan, R. (2022). Feasibility analysis of novel aerodynamic braking system for horizontal axis wind turbines. Materials Today: Proceedings, 68, 1396–1402. https://doi.org/10.1016/j.matpr.2022.06.444
- Lebid, V. H., Myrhorod, Yu. I. (2006). Aerohidrohazodynamika. Kharkiv: KhUPS, 350.
- Boyko, A. V., Usatiy, A. P., Rudenko, A. S. (2014). Mnogokriterial'naya mnogoparametricheskaya optimizatsiya protochnoy chasti osevyh turbin s uchetom rezhimov ekspluatatsii. Kharkiv: NTU «KhPI», 220. Available at: https://repository.kpi.kharkov.ua/server/api/core/bitstreams/0a708b9b-d194-40b9-9a83-1f92901459fa/content
- Veisi, A. A., Shafiei Mayam, M. H. (2017). Effects of blade rotation direction in the wake region of two in-line turbines using Large Eddy Simulation. Applied Energy, 197, 375–392. https://doi.org/10.1016/j.apenergy.2017.04.013
- Boiko, A., Govorushchenko, Y., Usaty, A. (2016). Optimization of the Axial Turbines Flow Paths. New York: Science Publishing Group, 272.
- Barbely, N. L., Komerath, N. M., Novak, L. A. (2016). A Study of Coaxial Rotor Performance and Flow Field Characteristics. AHS Technical Meeting on Aeromechanics Design for Vertical Lift. Report ARC-E-DAA-TN28910, 15. Available at: https://rotorcraft.arc.nasa.gov/Publications/files/CFD_Design_Framework_Barbely.pdf
- Ahmadi-Baloutaki, M., Carriveau, R., Ting, D. S.-K. (2016). A wind tunnel study on the aerodynamic interaction of vertical axis wind turbines in array configurations. Renewable Energy, 96, 904–913. https://doi.org/10.1016/j.renene.2016.05.060
- Simakov, N. N. (2013). Raschet obtekaniya i soprotivleniya shara v laminarnom i sil'no turbulentnom potoke. Zhurnal tehnicheskoy fiziki, 83 (4), 16–20.
- Papchenko, A., Lipovy, V., Barikin, O. (2013). Analysis of the airflow about sphere as a first approximation to determine the aerodynamic characteristics of airfoil profiles of orthogonal wind turbines. Visnyk Sumskoho derzhavnoho universytetu. Seriya Tekhnichni nauky, 1, 19–24. Available at: http://essuir.sumdu.edu.ua/handle/123456789/31937
- Rusanov, A. V., Ershov, S. V. (2008). Matematicheskoe modelirovanie nestatsionarnyh gazodinamicheskih protsessov v protochnyh chastyah turbomashin. Kharkiv: IPMash NAN Ukrainy, 275.
- Yershov, S. V., Rusanov, A. V., Yakovlev, V. A. (2006). Aerodynamic improvement of flowpath of the Hp turbine basing on computations of 3D viscous flow. Nauka ta innovatsiyi, 2 (6), 39–48. Available at: https://scinn.org.ua/sites/default/files/pdf/2006/N6/6_06_39.pdf
- Boiko, A., Usaty, A. P. (2017). Use BIarc-Curves for Contour Description of the Turbine Profiles. NTU “KhPI” Bulletin: Power and Heat Engineering Processes and Equipment, 8 (1230), 20–27. https://doi.org/10.20998/2078-774x.2017.08.03
- Lupoviy, V., Papchenko, A. (2014). Methods Development for Determining the Aerodynamic Characteristics of Vertical Exist of Wind Turbine. Applied Mechanics and Materials, 630, 79–84. https://doi.org/10.4028/www.scientific.net/amm.630.79
- Lipoviy, V., Papchenko, A. (2013). Research of workflow of vertical wind turbines by numerical simulation. Eastern-European Journal of Enterprise Technologies, 3 (8 (63), 39–44. Available at: https://journals.uran.ua/eejet/article/view/14834/
- Vion, L., Delattre, G., Falissard, F., Jacquin, L. (2011). Counter-Rotating Open Rotor (CROR): flow physics and simulation. CFM 2011 – 20th French Congress of Mechanics. Besancon, hal-03421272. Available at: https://hal.science/hal-03421272/document
- Tarasov, A. I. (2012). Izbrannye lektsii po kursu «Komp'yuternye tehnologi proektirovaniya baz dannyh» na temu «Postroenie reshetki turbinnyh profiley polinomami Bez'e-Bernshteyna». Kharkiv: NTU «KhPI», 27. Available at: https://www.kpi.kharkov.ua/archive/Articles/turbine/Построение%20решетки%20турбинных%20профилей%20.pdf
- Boiko, A., Usaty, A. P. (2016). Integrated Mathematical Model of the Processes in the Turbine with Adjustable Steam Extraction. NTU “KhPI” Bulletin: Power and Heat Engineering Processes and Equipment, 8 (1180), 28–36. https://doi.org/10.20998/2078-774x.2016.08.04
- Hmel'nik, S. I. (2010). Uravneniya Nav'e-Stoksa. Sushchestvovanie i metod poiska global'nogo resheniya. Published by Mathematics in Computer Corp., 106.
- Majstrenko, O. V., Prokopenko, V. V., Makeev, V. I., Ivanyk, E. G. (2020). Analytical methods of calculation of powered and passive trajectory of reactive and rocket-assisted projectiles. Radio Electronics, Computer Science, Control, 2, 173–182. https://doi.org/10.15588/1607-3274-2020-2-18
- Makeiev, V., Pushkariov, Y., Raskoshnyi, A., Voronko, I., Myronova, S. (2022). Considering the Meteorological Elements for the Aerial Vehicles Flight by Using “Weight” Functions. Lecture Notes in Networks and Systems, 133–144. https://doi.org/10.1007/978-3-030-94259-5_13
- Makeev, V. I., Pushkarev, Yu. I. (2013). Choice of working parameters of a solid-fuel jet engine of unguided aircraft. Russian Aeronautics (Iz VUZ), 56 (4), 344–353. https://doi.org/10.3103/s1068799813040041
- Nakaz komandyra v/ch A1723 vid 15.07.2021 roku No. 173/21.
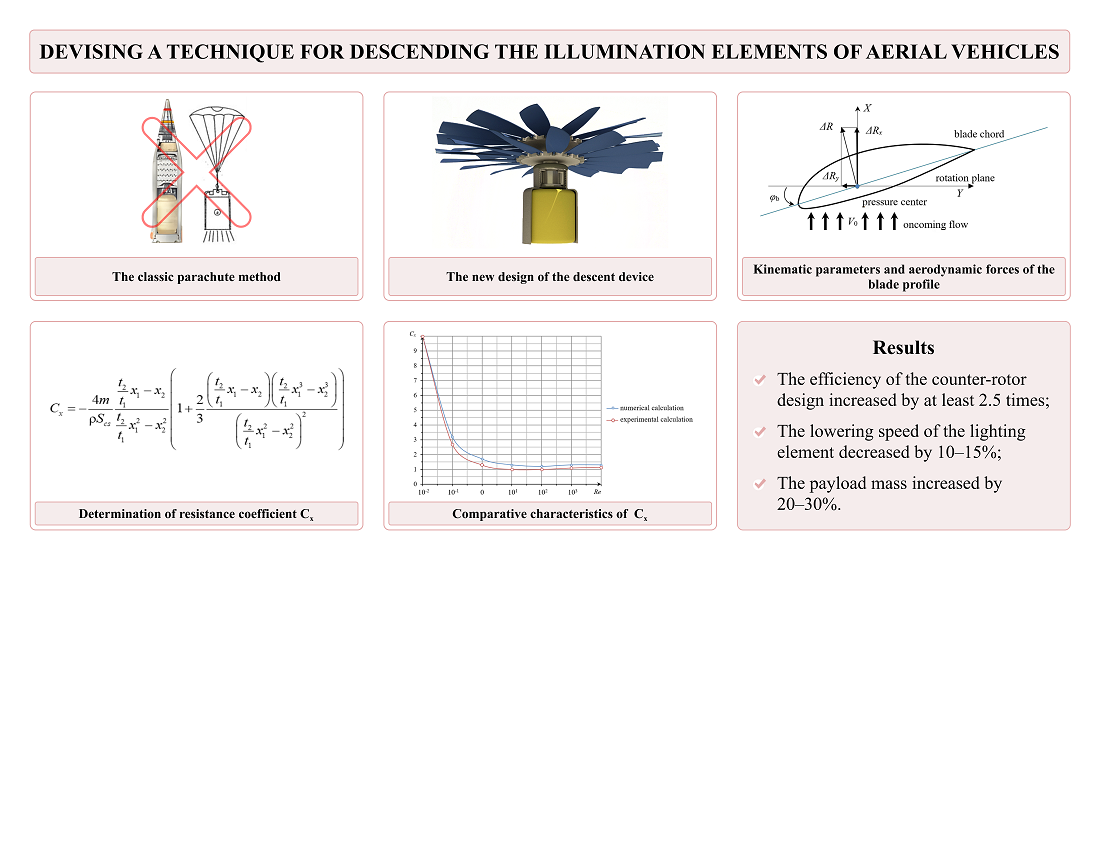
Downloads
Published
How to Cite
Issue
Section
License
Copyright (c) 2024 Vasyl Makeev, Anatoliy Derevjanchuk, Andrii Vakal, Mykola Liapa

This work is licensed under a Creative Commons Attribution 4.0 International License.
The consolidation and conditions for the transfer of copyright (identification of authorship) is carried out in the License Agreement. In particular, the authors reserve the right to the authorship of their manuscript and transfer the first publication of this work to the journal under the terms of the Creative Commons CC BY license. At the same time, they have the right to conclude on their own additional agreements concerning the non-exclusive distribution of the work in the form in which it was published by this journal, but provided that the link to the first publication of the article in this journal is preserved.
A license agreement is a document in which the author warrants that he/she owns all copyright for the work (manuscript, article, etc.).
The authors, signing the License Agreement with TECHNOLOGY CENTER PC, have all rights to the further use of their work, provided that they link to our edition in which the work was published.
According to the terms of the License Agreement, the Publisher TECHNOLOGY CENTER PC does not take away your copyrights and receives permission from the authors to use and dissemination of the publication through the world's scientific resources (own electronic resources, scientometric databases, repositories, libraries, etc.).
In the absence of a signed License Agreement or in the absence of this agreement of identifiers allowing to identify the identity of the author, the editors have no right to work with the manuscript.
It is important to remember that there is another type of agreement between authors and publishers – when copyright is transferred from the authors to the publisher. In this case, the authors lose ownership of their work and may not use it in any way.