Optimization of distributed acoustic sensors based on fiber optic technologies
DOI:
https://doi.org/10.15587/1729-4061.2024.313455Keywords:
fiber optic technologies, distributed acoustic sensors, seismic monitoring, infrastructure monitoringAbstract
This research investigates distributed acoustic sensors (DAS) based on fiber optic technologies, focusing on the impact of pressure on signal-to-noise ratio (SNR), noise levels, and dominant frequency shifts. DAS systems are widely used for infrastructure monitoring due to their ability to capture acoustic signals over long distances, making them ideal for seismic and pipeline monitoring.
The study examines how fluctuating pressure affects DAS performance, particularly signal quality and noise reduction. In applications like pipeline leak detection and seismic monitoring, pressure changes can degrade signal clarity and complicate anomaly detection. Understanding this relationship is key to optimizing DAS performance and improving system efficiency.
The experiment varied pressure from 0.1 atm to 5 atm, showing that increased pressure raised SNR from 10 dB to 48 dB, reduced noise from 10 dB to 7 dB, and shifted the dominant frequency from 0.5 Hz to 3 Hz. Fourier analysis provided insights into these frequency spectrum changes. Higher pressure compresses the medium, enhancing signal isolation and improving SNR while reducing noise. The frequency shift results from changes in acoustic wave propagation speed under higher pressure, highlighting its role in signal processing.
The key finding is that higher pressure significantly improves signal quality and reduces noise, enhancing DAS performance. The frequency shift improves environmental detection capabilities. These results are valuable for DAS applications in environments with pressure variations, like pipeline monitoring, where high signal quality is crucial. Improved signal fidelity and frequency shifts make DAS systems more reliable for long-term monitoring and contribute to accurate anomaly detection
References
- Udd, E., Spillman, W. B. (Eds.) (2024). Fiber Optic Sensors. John Wiley & Sons, Inc. https://doi.org/10.1002/9781119678892
- Ashry, I., Mao, Y., Wang, B., Hveding, F., Bukhamsin, A., Ng, T. K., Ooi, B. S. (2022). A Review of Distributed Fiber–Optic Sensing in the Oil and Gas Industry. Journal of Lightwave Technology, 40 (5), 1407–1431. https://doi.org/10.1109/jlt.2021.3135653
- Hveding, F., Bukhamsin, A. (2018). Distributed Fiber Optic Sensing – A Technology Review for Upstream Oil and Gas Applications. All Days. https://doi.org/10.2118/192323-ms
- Mikhailov, P., Ualiyev, Z., Kabdoldina, A., Smailov, N., Khikmetov, A., Malikova, F. (2021). Multifunctional fiber-optic sensors for space infrastructure. Eastern-European Journal of Enterprise Technologies, 5 (5 (113)), 80–89. https://doi.org/10.15587/1729-4061.2021.242995
- Sekenov, B., Smailov, N., Tashtay, Y., Amir, A., Kuttybayeva, A., Tolemanova, A. (2024). Fiber-Optic Temperature Sensors for Monitoring the Influence of the Space Environment on Nanosatellites: A Review. Advances in Asian Mechanism and Machine Science, 371–380. https://doi.org/10.1007/978-3-031-67569-0_42
- Khabay, A., Baktybayev, M., Ibekeyev, S., Sarsenbayev, N., Junussov, N., Zhumakhan, N. (2024). Improvement of fiber optic sensor measurement methods for temperature and humidity measurement in microelectronic circuits. Eastern-European Journal of Enterprise Technologies, 3 (5 (129)), 36–44. https://doi.org/10.15587/1729-4061.2024.306711
- Parker, T., Shatalin, S., Farhadiroushan, M. (2014). Distributed Acoustic Sensing – a new tool for seismic applications. First Break, 32 (2). https://doi.org/10.3997/1365-2397.2013034
- Masoudi, A., Newson, T. P. (2016). Contributed Review: Distributed optical fibre dynamic strain sensing. Review of Scientific Instruments, 87 (1). https://doi.org/10.1063/1.4939482
- Hartog, A. H. (2017). An Introduction to Distributed Optical Fibre Sensors. CRC Press. https://doi.org/10.1201/9781315119014
- Gonzalez-Herraez, M., Fernandez-Ruiz, M. R., Magalhaes, R., Costa, L., Martins, H. F., Becerril, C. et al. (2021). Distributed Acoustic Sensing for Seismic Monitoring. Optical Fiber Communication Conference (OFC) 2021, 9, Tu1L.2. https://doi.org/10.1364/ofc.2021.tu1l.2
- Dou, S., Lindsey, N., Wagner, A. M., Daley, T. M., Freifeld, B., Robertson, M. et al. (2017). Distributed Acoustic Sensing for Seismic Monitoring of The Near Surface: A Traffic-Noise Interferometry Case Study. Scientific Reports, 7 (1). https://doi.org/10.1038/s41598-017-11986-4
- Zhu, H.-H., Liu, W., Wang, T., Su, J.-W., Shi, B. (2022). Distributed Acoustic Sensing for Monitoring Linear Infrastructures: Current Status and Trends. Sensors, 22 (19), 7550. https://doi.org/10.3390/s22197550
- Martins, W. A., de Campos, M. L. R., da Silva Chaves, R., Lordelo, C. P. V., Ellmauthaler, A., Nunes, L. O., Barfoot, D. A. (2017). Communication Models for Distributed Acoustic Sensing for Telemetry. IEEE Sensors Journal, 17 (15), 4677–4688. https://doi.org/10.1109/jsen.2017.2714023
- Wang, Y., Yuan, H., Liu, X., Bai, Q., Zhang, H., Gao, Y., Jin, B. (2019). A Comprehensive Study of Optical Fiber Acoustic Sensing. IEEE Access, 7, 85821–85837. https://doi.org/10.1109/access.2019.2924736
- Cannon, R., Aminzadeh, F. (2013). Distributed Acoustic Sensing: State of the Art. All Days. https://doi.org/10.2118/163688-ms
- Soroush, M., Mohammadtabar, M., Roostaei, M., Hosseini, S. A., Fattahpour, V., Mahmoudi, M. et al. (2022). Downhole Monitoring Using Distributed Acoustic Sensing: Fundamentals and Two Decades Deployment in Oil and Gas Industries. Day 3 Wed, March 23, 2022. https://doi.org/10.2118/200088-ms
- Johannessen, K., Drakeley, B., Farhadiroushan, M. (2012). Distributed Acoustic Sensing - A New Way of Listening to Your Well/Reservoir. All Days. https://doi.org/10.2118/149602-ms
- Lindsey, N. J., Martin, E. R., Dreger, D. S., Freifeld, B., Cole, S., James, S. R. et al. (2017). Fiber‐Optic Network Observations of Earthquake Wavefields. Geophysical Research Letters, 44 (23). https://doi.org/10.1002/2017gl075722
- Zhan, Z. (2019). Distributed Acoustic Sensing Turns Fiber‐Optic Cables into Sensitive Seismic Antennas. Seismological Research Letters, 91 (1), 1–15. https://doi.org/10.1785/0220190112
- Sladen, A., Rivet, D., Ampuero, J. P., De Barros, L., Hello, Y., Calbris, G., Lamare, P. (2019). Distributed sensing of earthquakes and ocean-solid Earth interactions on seafloor telecom cables. Nature Communications, 10 (1). https://doi.org/10.1038/s41467-019-13793-z
- Murayama, H., Wada, D., Igawa, H. (2013). Structural health monitoring by using fiber-optic distributed strain sensors with high spatial resolution. Photonic Sensors, 3 (4), 355–376. https://doi.org/10.1007/s13320-013-0140-5
- Eum, S. H., Kageyama, K., Murayama, H., Uzawa, K., Ohsawa, I., Kanai, M. et al. (2007). Structural health monitoring using fiber optic distributed sensors for vacuum-assisted resin transfer molding. Smart Materials and Structures, 16 (6), 2627–2635. https://doi.org/10.1088/0964-1726/16/6/067
- Fan, X., He, Z., Liu, Q., Chen, D., Wang, S., Yang, G. (2018). Fiber-optic distributed acoustic sensors (DAS) and applications in railway perimeter security. Advanced Sensor Systems and Applications VIII, 28, 1. https://doi.org/10.1117/12.2505342
- Ružička, M., Münster, P., Dejdar, P., Jablončík, L. (2021). Distributed optical fiber acoustic sensing system for perimeter security. Security & Future, 5 (4), 150–152. Available at: https://stumejournals.com/journals/confsec/2021/4/150.full.pdf
- Kabdoldina, A., Ualiyev, Z., Smailov, N., Malikova, F., Oralkanova, K., Baktybayev, M. et al. (2022). Development of the design and technology for manufacturing a combined fiber-optic sensor used for extreme operating conditions. Eastern-European Journal of Enterprise Technologies, 5 (5 (119)), 34–43. https://doi.org/10.15587/1729-4061.2022.266359
- Smailov, N., Zhadiger, T., Tashtay, Y., Abdykadyrov, A., Amir, A. (2024). Fiber laser-based two-wavelength sensors for detecting temperature and strain on concrete structures. International Journal of Innovative Research and Scientific Studies, 7 (4), 1693–1710. https://doi.org/10.53894/ijirss.v7i4.3481
- Kuttybayeva, A., Sabibolda, A., Kengesbayeva, S., Baigulbayeva, M., Amir, A., Sekenov, B. (2024). Investigation of a Fiber Optic Laser Sensor with Grating Resonator Using Mirrors. 2024 Conference of Young Researchers in Electrical and Electronic Engineering (ElCon). https://doi.org/10.1109/elcon61730.2024.10468264
- Sabibolda, A., Tsyporenko, V., Smailov, N., Tsyporenko, V., Abdykadyrov, A. (2024). Estimation of the Time Efficiency of a Radio Direction Finder Operating on the Basis of a Searchless Spectral Method of Dispersion-Correlation Radio Direction Finding. Advances in Asian Mechanism and Machine Science, 62–70. https://doi.org/10.1007/978-3-031-67569-0_8
- Smailov, N., Tsyporenko, V., Sabibolda, A., Tsyporenko, V., Kabdoldina, A., Zhekambayeva, M. et al. (2023). Improving the accuracy of a digital spectral correlation-interferometric method of direction finding with analytical signal reconstruction for processing an incomplete spectrum of the signal. Eastern-European Journal of Enterprise Technologies, 5 (9 (125)), 14–25. https://doi.org/10.15587/1729-4061.2023.288397
- Sabibolda, A., Tsyporenko, V., Tsyporenko, V., Smailov, N., Zhunussov, K., Abdykadyrov, A. et al. (2022). Improving the accuracy and performance speed of the digital spectral-correlation method for measuring delay in radio signals and direction finding. Eastern-European Journal of Enterprise Technologies, 1 (9(115)), 6–14. https://doi.org/10.15587/1729-4061.2022.252561
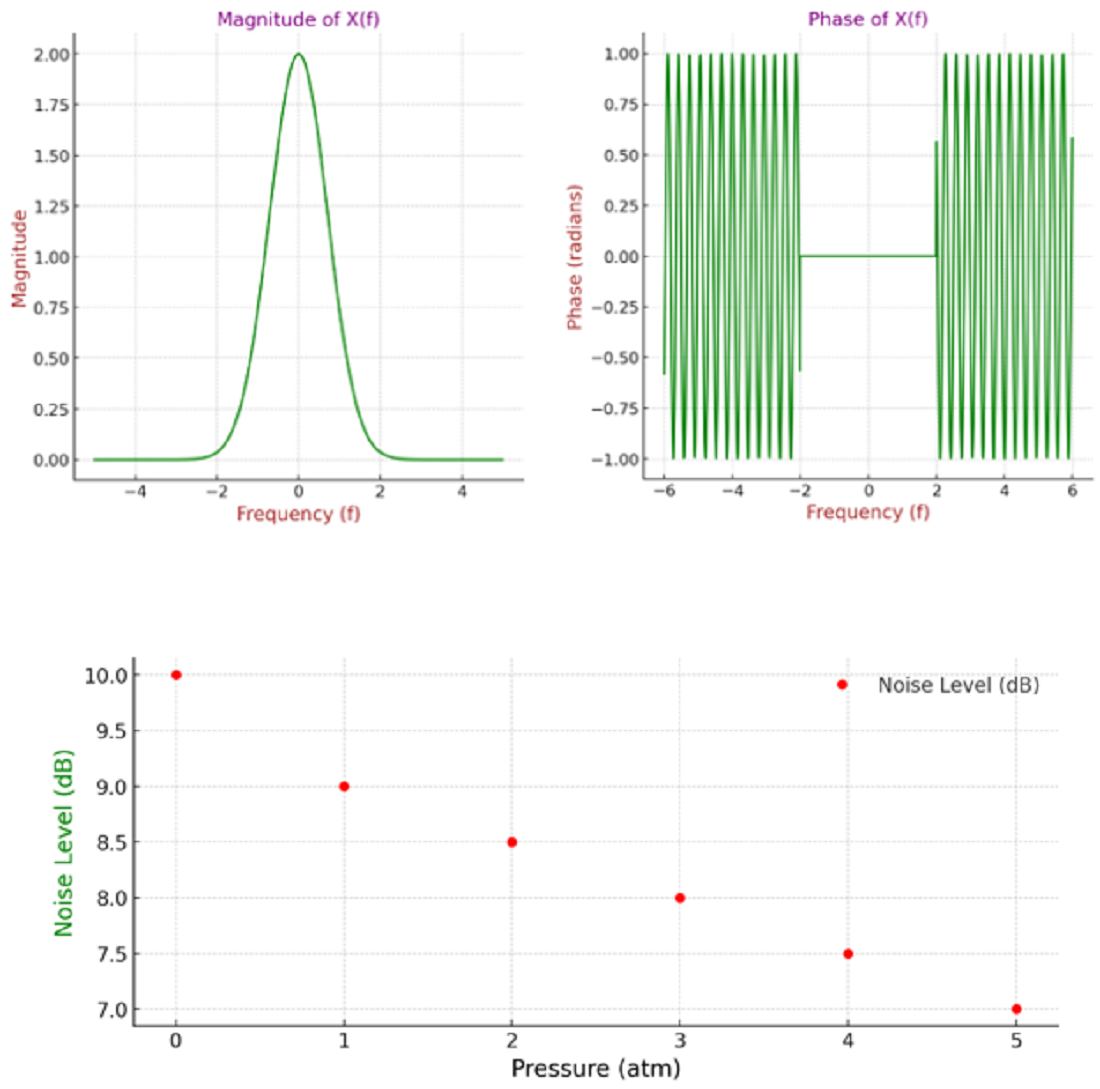
Downloads
Published
How to Cite
Issue
Section
License
Copyright (c) 2024 Askar Abdykadyrov, Nurzhigit Smailov, Akezhan Sabibolda, Gulzhaina Tolen, Zhandos Dosbayev, Zhomart Ualiyev, Rashida Kadyrova

This work is licensed under a Creative Commons Attribution 4.0 International License.
The consolidation and conditions for the transfer of copyright (identification of authorship) is carried out in the License Agreement. In particular, the authors reserve the right to the authorship of their manuscript and transfer the first publication of this work to the journal under the terms of the Creative Commons CC BY license. At the same time, they have the right to conclude on their own additional agreements concerning the non-exclusive distribution of the work in the form in which it was published by this journal, but provided that the link to the first publication of the article in this journal is preserved.
A license agreement is a document in which the author warrants that he/she owns all copyright for the work (manuscript, article, etc.).
The authors, signing the License Agreement with TECHNOLOGY CENTER PC, have all rights to the further use of their work, provided that they link to our edition in which the work was published.
According to the terms of the License Agreement, the Publisher TECHNOLOGY CENTER PC does not take away your copyrights and receives permission from the authors to use and dissemination of the publication through the world's scientific resources (own electronic resources, scientometric databases, repositories, libraries, etc.).
In the absence of a signed License Agreement or in the absence of this agreement of identifiers allowing to identify the identity of the author, the editors have no right to work with the manuscript.
It is important to remember that there is another type of agreement between authors and publishers – when copyright is transferred from the authors to the publisher. In this case, the authors lose ownership of their work and may not use it in any way.