Development of a mathematical model for the cooling channel of a liquid propellant rocket engine’s chamber with respect for variations in coolant density
DOI:
https://doi.org/10.15587/1729-4061.2024.316236Keywords:
liquid propellant rocket engine, mathematical model of cooling channels, propellant density variationAbstract
This study focuses on the processes occurring within the cooling channels of a liquid rocket engine chamber. It is important to note that, from a design-stage efficiency prediction perspective, the cooling system is the most critical part of the engine chamber. This is because it cannot be tested without costly and labor-intensive fire tests. Therefore, mathematical models of heat transfer and fluid dynamics must describe accurately all processes taking place in the chamber.
The study emphasizes accounting for the change in the density of the propellant component within the cooling channels. To verify the importance of this issue, an analysis was conducted on the changing parameters of the propellant components in the cooling channels of an engine. The assessment revealed that even when using high-boiling propellant components and moderate heating in the cooling channels, density changes can exceed 25 %.
This paper presents the results of developing a model for the flow of propellant in the cooling channels of a liquid rocket engine chamber, taking density changes into account. The model builds on a cooling channel model previously developed by the authors. An equation that accounts for density variations was derived using established principles of fluid mechanics.
Using the developed mathematical model, test calculations were performed, and the simulation results were compared with and without considering density changes. Furthermore, a comparison was conducted with calculated data available in the literature on heat transfer in the RD107 engine chamber, revealing an error margin of no more than 1.5 %.
The resulting mathematical model may be recommended for use in the design of new rocket engine chambers with regenerative cooling
References
- Arnold, S. (1999). Physical & Thermodynamic Properties of Hypergolic Propellants: A Review and Update. Conference: JANNAF Inter-agency Propulsion Committee PD&CS and S&EPS Joint Meeting.
- Nesterenko, V. (1982). Teplofizicheskie svoystva chetyrekhokisi azota. Minsk: Nauka i tekhnika, 197.
- Sliusariev, V., Bucharskyi, V. (2024). Development of a differential model for cooling an LPRE chamber by an incompressible fluid. Journal of Rocket-Space Technology, 33 (4 (28)), 49–58. https://doi.org/10.15421/452424
- Dubrovskiy, I., Bucharskyi, V. (2023). Devising a method to design supersonic nozzles of rocket engines by using numerical analysis methods. Eastern-European Journal of Enterprise Technologies, 6 (1 (126)), 61–67. https://doi.org/10.15587/1729-4061.2023.290583
- Dubrovskiy, I., Bucharskyi, V. (2020). Development of a method of extended cells for the formulation of boundary conditions in numerical integration of gas dynamics equations in the domains of a curvilinear shape. Eastern-European Journal of Enterprise Technologies, 5 (7 (107)), 74–82. https://doi.org/10.15587/1729-4061.2020.213795
- Bucharskyi, V., Zhang, L.-H., Wan, Y.-L. (2018). Improvement in Time Efficiency in Numerical Simulation for Solid Propellant Rocket Motors (SPRM). Journal of Propulsion Technology, 39 (1), 92–99. https://doi.org/10.13675/j.cnki.tjjs.2018.01.010
- Sichler, E., Montes, J. D., Chandler, F. O. (2018). One Dimensional Thermal Steady State Analysis and Procedure for a Low-Pressure Liquid Oxygen and Liquid Methane Rocket Engine. 2018 Joint Propulsion Conference. https://doi.org/10.2514/6.2018-4602
- Kose, Y. M., Celik, M. (2023). Regenerative Cooling Comparison of LOX/LCH4 and LOX/LC3H8 Rocket Engines Using the One-Dimensional Regenerative Cooling Modelling Tool ODREC. Applied Sciences, 14 (1), 71. https://doi.org/10.3390/app14010071
- Bergman, T., Lavine, A., Incropera, F., DeWitt, D. (2011). Fundamentals of Heat and Mass Transfer. Hoboken: John Wiley & Sons, Inc.
- Lemmon, E., Huber, M., McLinden, M. (2013). NIST Standard Reference Database 23: Reference Fluid Thermodynamic and Transport Properties-REFPROP, Version 9.1. Available at: https://www.nist.gov/publications/nist-standard-reference-database-23-reference-fluid-thermodynamic-and-transport
- Song, J., Liang, T., Li, Q., Cheng, P., Zhang, D., Cui, P., Sun, J. (2021). Study on the heat transfer characteristics of regenerative cooling for LOX/LCH4 variable thrust rocket engine. Case Studies in Thermal Engineering, 28, 101664. https://doi.org/10.1016/j.csite.2021.101664
- Lv, J., Du, G., Jin, P., Li, R. (2023). Heat Transfer Analysis and Structural Optimization for Spiral Channel Regenerative Cooling Thrust Chamber. International Journal of Aerospace Engineering, 2023, 1–17. https://doi.org/10.1155/2023/8628107
- Atefi, E., Naraghi, M. H. (2019). Optimization of Regeneratively Cooled Rocket Engines Cooling Channel Dimensions. AIAA Propulsion and Energy 2019 Forum. https://doi.org/10.2514/6.2019-3938
- NIST Standard Reference Database 4. NIST. Available at: https://www.nist.gov/srd/nist-standard-reference-database-4
- Li, X., Wu, S., Zhang, Q., Li, X., Chen, S. (2024). A novel method based on the calculus of variations to optimize the cooling passage configuration in thermal protection structure. Journal of Physics: Conference Series, 2764 (1), 012038. https://doi.org/10.1088/1742-6596/2764/1/012038
- Fagherazzi, M., Santi, M., Barato, F., Pizzarelli, M. (2023). A Simplified Thermal Analysis Model for Regeneratively Cooled Rocket Engine Thrust Chambers and Its Calibration with Experimental Data. Aerospace, 10 (5), 403. https://doi.org/10.3390/aerospace10050403
- Romano, A., Ricci, D., Battista, F. (2024). 1D numerical simulations aimed to reproduce the operative conditions of a LOX/LCH4 engine demonstrator. https://doi.org/10.21203/rs.3.rs-3866302/v1
- Kim, S.-K., Joh, M., Choi, H. S., Park, T. S. (2014). Effective Modeling of Conjugate Heat Transfer and Hydraulics for the Regenerative Cooling Design of Kerosene Rocket Engines. Numerical Heat Transfer, Part A: Applications, 66 (8), 863–883. https://doi.org/10.1080/10407782.2014.892396
- Jeong, W., Jang, S., Kim, H.-J. (2023). Characteristics of a Heat Exchanger in a Liquid Rocket Engine Using Conjugate Heat Transfer Coupling with Open-Source Tools. Aerospace, 10 (12), 983. https://doi.org/10.3390/aerospace10120983
- Xu, B., Chen, B., Peng, J., Zhou, W., Xu, X. (2023). A Coupled Heat Transfer Calculation Strategy for Composite Cooling Liquid Rocket Engine. Aerospace, 10 (5), 473. https://doi.org/10.3390/aerospace10050473
- Jin, X., Shen, C., Wu, X. (2020). Numerical Study on Regenerative Cooling Characteristics of Kerosene Scramjets. International Journal of Aerospace Engineering, 2020, 1–12. https://doi.org/10.1155/2020/8813929
- Belyaev, E. (1987). Termodinamika. Kyiv: «Vischa shkola», 344.
- ZHRD RD-107 i RD-108 i ih modifikatsii. Liquid Propellant Rocket Engines. Available at: http://www.lpre.de/energomash/RD-107/index.htm
- Colebrook, C. F. (1939). Turbulent flow in pipes, with particular reference to the transition region between the smooth and rough pipe laws. Journal of the Institution of Civil Engineers, 11 (4), 133–156. https://doi.org/10.1680/ijoti.1939.13150
- Stimpson, C. K., Snyder, J. C., Thole, K. A., Mongillo, D. (2016). Scaling Roughness Effects on Pressure Loss and Heat Transfer of Additively Manufactured Channels. Journal of Turbomachinery, 139 (2). https://doi.org/10.1115/1.4034555
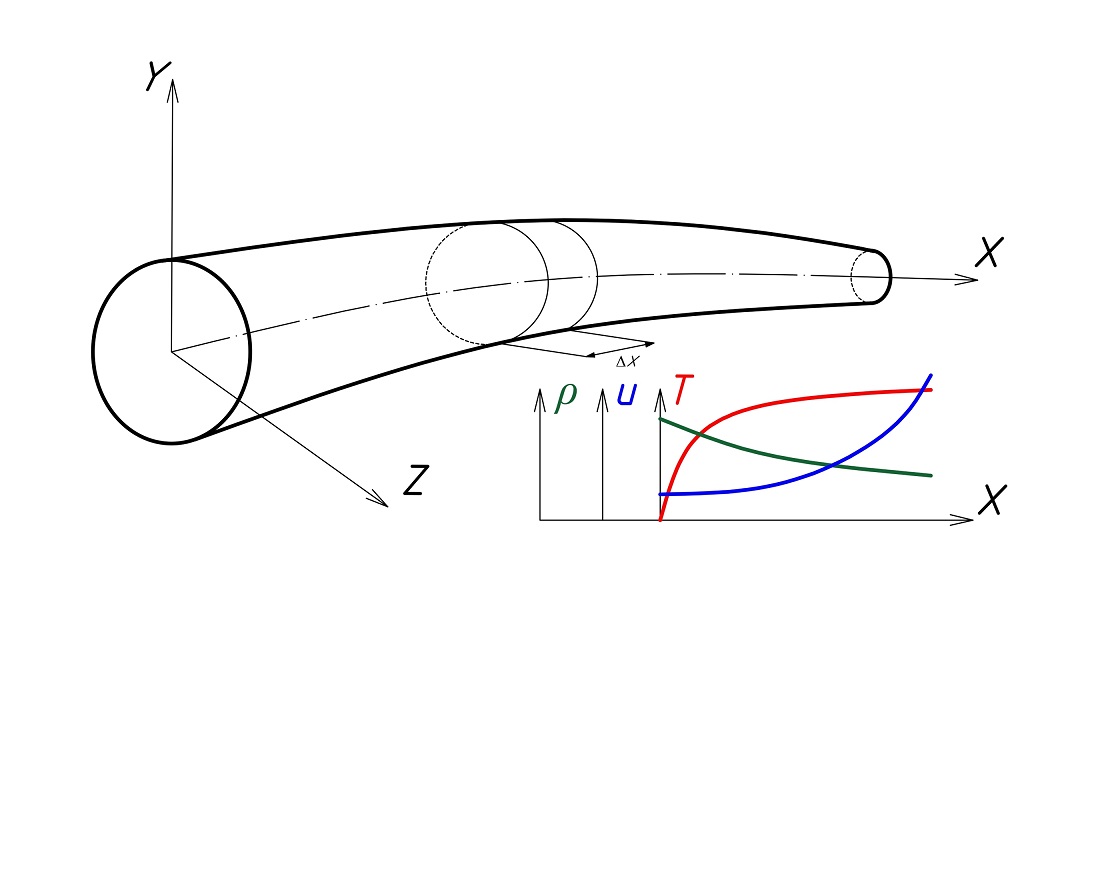
Downloads
Published
How to Cite
Issue
Section
License
Copyright (c) 2024 Volodymyr Sliusariev, Valeriy Bucharskyi

This work is licensed under a Creative Commons Attribution 4.0 International License.
The consolidation and conditions for the transfer of copyright (identification of authorship) is carried out in the License Agreement. In particular, the authors reserve the right to the authorship of their manuscript and transfer the first publication of this work to the journal under the terms of the Creative Commons CC BY license. At the same time, they have the right to conclude on their own additional agreements concerning the non-exclusive distribution of the work in the form in which it was published by this journal, but provided that the link to the first publication of the article in this journal is preserved.
A license agreement is a document in which the author warrants that he/she owns all copyright for the work (manuscript, article, etc.).
The authors, signing the License Agreement with TECHNOLOGY CENTER PC, have all rights to the further use of their work, provided that they link to our edition in which the work was published.
According to the terms of the License Agreement, the Publisher TECHNOLOGY CENTER PC does not take away your copyrights and receives permission from the authors to use and dissemination of the publication through the world's scientific resources (own electronic resources, scientometric databases, repositories, libraries, etc.).
In the absence of a signed License Agreement or in the absence of this agreement of identifiers allowing to identify the identity of the author, the editors have no right to work with the manuscript.
It is important to remember that there is another type of agreement between authors and publishers – when copyright is transferred from the authors to the publisher. In this case, the authors lose ownership of their work and may not use it in any way.