Creation of a distributed energy system for the production of thermal and electric energy
DOI:
https://doi.org/10.15587/1729-4061.2024.318785Keywords:
cogeneration distributed generation, renewable energy sources, off-grid energy, biogas, electrical and thermal energyAbstract
The object of the study is the distributed generation (DG) system for remote areas where extending power lines is challenging or impossible. The study demonstrates how integrating electrical and thermal energy modules based on renewable energy sources (RES) into a common DG bus can ensure continuous energy supply. This approach provides both heat and electricity to consumers, independent of weather conditions an advantage over traditional systems reliant on variable sources like wind and solar energy. Numerical assessments suggest that the proposed system can improve local renewable resource utilization by approximately 20–30 % compared to single-source renewable setups. This enhanced efficiency results in a more stable power output, with fewer interruptions caused by low wind speeds or reduced solar irradiance. Economically, reducing dependence on diesel generators by about 15–25 % can translate into substantial fuel cost savings. In addition, shifting energy production away from non-renewable sources may cut greenhouse gas emissions by an estimated 10–20 %, contributing to environmental protection targets. In this research received lies in its solution for off-grid energy delivery in rural areas, which generally rely on expensive and frequently unreliable centralized energy infrastructure. By leveraging renewable energy sources and implementing a cogenerative DG system, the study significantly reduces reliance on traditional energy grids and enhances energy independence for remote facilities. The research highlights the practical value of the proposed solution, particularly for rural areas far from power lines and with limited access to traditional electricity systems. The suggested system not only provides continuous energy, but it also coincides with worldwide trends toward sustainable and decentralized energy solutions
References
- Fu, X., Wei, Z., Sun, H., Zhang, Y. (2024). Agri-Energy-Environment Synergy-Based Distributed Energy Planning in Rural Areas. IEEE Transactions on Smart Grid, 15 (4), 3722–3738. https://doi.org/10.1109/tsg.2024.3364182
- Rustamov, N., Babakhan, S., Genc, N., Kibishov, A., Meirbekova, O. (2023). An Improved Hybrid Wind Power Plant for Small Power Generation. International Journal of Renewable Energy Research, 13 (2). https://doi.org/10.20508/ijrer.v13i2.14193.g8735
- Rustamov, N., Meirbekova, O., Kibishov, А., Babakhan, S., Berguzinov, А. (2022). Creation of a hybrid power plant operating on the basis of a gas turbine engine. Eastern-European Journal of Enterprise Technologies, 2 (8 (116)), 29–37. https://doi.org/10.15587/1729-4061.2022.255451
- Zhou, Y., Wang, J., Xu, H., Yang, M., Liu, W. (2024). Improving full-chain process synergy of multi-energy complementary distributed energy system in cascade storage and initiative management strategies. Energy Conversion and Management, 322, 119120. https://doi.org/10.1016/j.enconman.2024.119120
- Tahir, M. F., Haoyong, C., Mehmood, K., Ali, N., Bhutto, J. A. (2019). Integrated Energy System Modeling of China for 2020 by Incorporating Demand Response, Heat Pump and Thermal Storage. IEEE Access, 7, 40095–40108. https://doi.org/10.1109/access.2019.2905684
- Li, C., Yang, H., Shahidehpour, M., Xu, Z., Zhou, B., Cao, Y., Zeng, L. (2020). Optimal Planning of Islanded Integrated Energy System With Solar-Biogas Energy Supply. IEEE Transactions on Sustainable Energy, 11 (4), 2437–2448. https://doi.org/10.1109/tste.2019.2958562
- Giordano, A., Mastroianni, C., Menniti, D., Pinnarelli, A., Scarcello, L., Sorrentino, N. (2021). A Two-Stage Approach for Efficient Power Sharing Within Energy Districts. IEEE Transactions on Systems, Man, and Cybernetics: Systems, 51 (3), 1679–1689. https://doi.org/10.1109/tsmc.2019.2902077
- Nadeem, F., Hussain, S. M. S., Tiwari, P. K., Goswami, A. K., Ustun, T. S. (2019). Comparative Review of Energy Storage Systems, Their Roles, and Impacts on Future Power Systems. IEEE Access, 7, 4555–4585. https://doi.org/10.1109/access.2018.2888497
- Zhang, Y., Yuan, F., Zhai, H., Song, C., Poursoleiman, R. (2023). RETRACTED: Optimizing the planning of distributed generation resources and storages in the virtual power plant, considering load uncertainty. Journal of Cleaner Production, 387, 135868. https://doi.org/10.1016/j.jclepro.2023.135868
- Niknam, T., Kavousi-Fard, A., Ostadi, A. (2015). Impact of Hydrogen Production and Thermal Energy Recovery of PEMFCPPs on Optimal Management of Renewable Microgrids. IEEE Transactions on Industrial Informatics, 11 (5), 1190–1197. https://doi.org/10.1109/tii.2015.2475715
- Ariwoola, R., Kamalasadan, S. (2023). An Integrated Hybrid Thermal Dynamics Model and Energy Aware Optimization Framework for Grid-Interactive Residential Building Management. IEEE Transactions on Industry Applications, 59 (2), 2519–2531. https://doi.org/10.1109/tia.2022.3224689
- Tian, Z., Li, X., Niu, J., Zhou, R., Li, F. (2024). Enhancing operation flexibility of distributed energy systems: A flexible multi-objective optimization planning method considering long-term and temporary objectives. Energy, 288, 129612. https://doi.org/10.1016/j.energy.2023.129612
- Xie, H., Ahmad, T., Zhang, D., Goh, H. H., Wu, T. (2024). Community-based virtual power plants’ technology and circular economy models in the energy sector: A Techno-economy study. Renewable and Sustainable Energy Reviews, 192, 114189. https://doi.org/10.1016/j.rser.2023.114189
- Evro, S., Oni, B. A., Tomomewo, O. S. (2024). Carbon neutrality and hydrogen energy systems. International Journal of Hydrogen Energy, 78, 1449–1467. https://doi.org/10.1016/j.ijhydene.2024.06.407
- Zheng, Z., Shafique, M., Luo, X., Wang, S. (2024). A systematic review towards integrative energy management of smart grids and urban energy systems. Renewable and Sustainable Energy Reviews, 189, 114023. https://doi.org/10.1016/j.rser.2023.114023
- Rustamov, N. T., Mejrbekov, A. T., Meirbekova, D. (2022). Pat. No. 29833 RK. Method of all-season power supply to a greenhouse from an alternative energy source. publ.: 04.01.2022.
- Karbowa, K., Wnukowska, B., Czosnyka, M. (2019). Computer Aided Selection Of Power Generation unit In The Cogeneration Process. 2019 Progress in Applied Electrical Engineering (PAEE), 1–7. https://doi.org/10.1109/paee.2019.8788998
- Dyussebayev, I. M., Issabekov, Zh., Tulegulov, A. D., Yergaliyev, D. S., Bazhaev, N. A., Kaipova, A. A. (2022). Methodological basis for the application of wind generators in geology. Series Of Geology And Technical Sciences, 5 (455), 63–78. https://doi.org/10.32014/2518-170x.218
- Rustamov, N. T., Meirbekov, A. T., Avezova, N. R., Meirbekova, O. D., Babakhan, Sh. A. (2022). Pat. No. 7970 RK. Hybrid system for generating thermal and electrical energy. publ.: 24.11.2022.
- Diaz-Cachinero, P., Munoz-Hernandez, J. I., Contreras, J. (2018). A Linear Model for Operating Microgrids with Renewable Resources, Battery Degradation Costs and Electric Vehicles. 2018 15th International Conference on the European Energy Market (EEM), 1–5. https://doi.org/10.1109/eem.2018.8469868
- Bramm, A. M., Matrenin, P. V., Papkova, N. A., Sekatski, D. A. (2024). Capacity Factor Forecasting for Generation Facilities Based on Renewable Energy Sources in Decentralized Power Systems. ENERGETIKA. Proceedings of CIS Higher Education Institutions and Power Engineering Associations, 67 (5), 411–424. https://doi.org/10.21122/1029-7448-2024-67-5-411-424
- Sultanov, M. M., Arakelyan, E. K., Boldyrev, I. A., Lunenko, V. S., Menshikov, P. D. (2021). Digital twins application in control systems for distributed generation of heat and electric energy. Archives of Thermodynamics, 42 (2), 89–101. https://doi.org/10.24425/ather.2021.137555
- Boghdady, T., Sweed, I. A., Ibrahim, D. K. (2023). Performance Enhancement of Doubly-Fed Induction Generator-Based-Wind Energy System. International Journal of Renewable Energy Research, 13 (1). https://doi.org/10.20508/ijrer.v13i1.13649.g8685
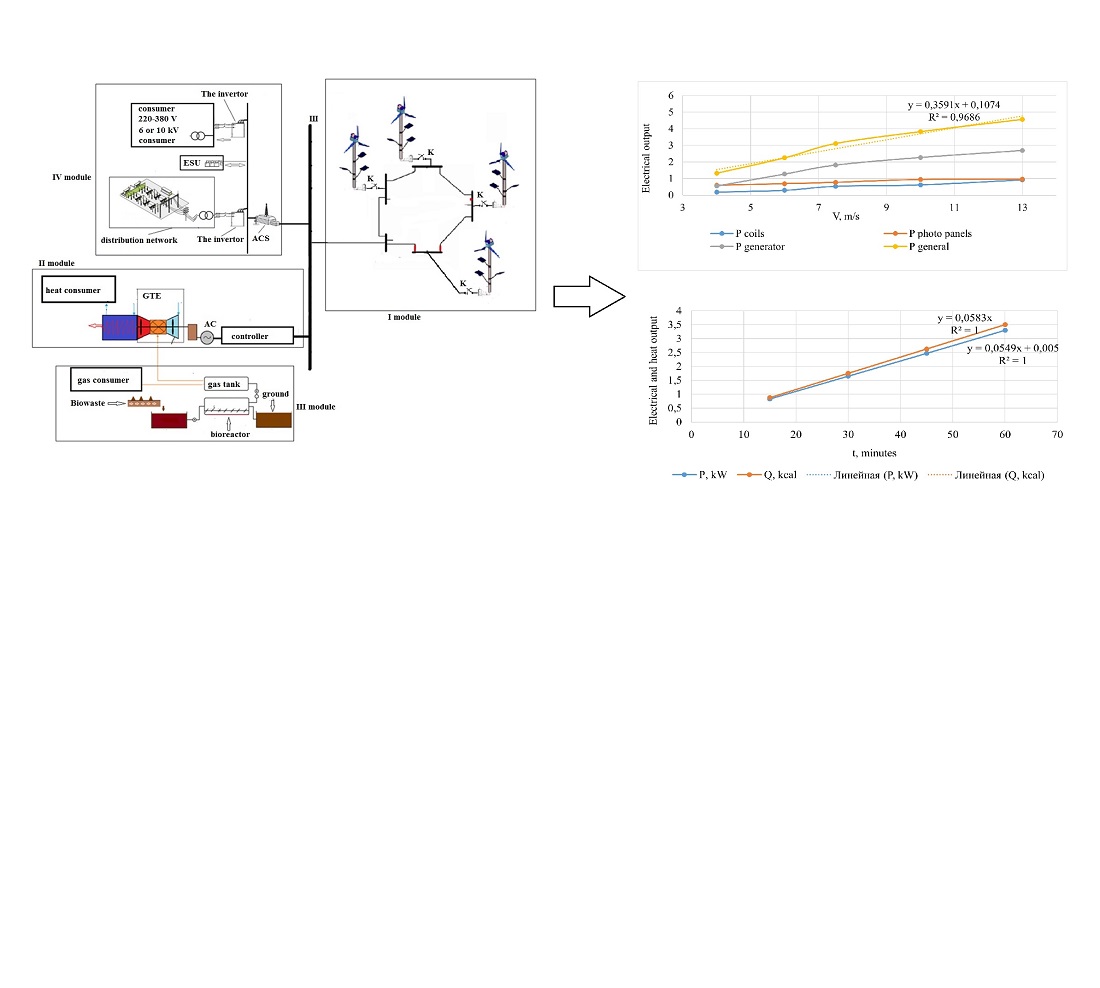
Downloads
Published
How to Cite
Issue
Section
License
Copyright (c) 2024 Nassim Rustamov, Kamalbek Berkimbayev, Zagipa Abdikulova, Oxana Meirbekova, Zhanibek Issabekov, Shokhrukh Babakhan, Perizat Rakhmetova

This work is licensed under a Creative Commons Attribution 4.0 International License.
The consolidation and conditions for the transfer of copyright (identification of authorship) is carried out in the License Agreement. In particular, the authors reserve the right to the authorship of their manuscript and transfer the first publication of this work to the journal under the terms of the Creative Commons CC BY license. At the same time, they have the right to conclude on their own additional agreements concerning the non-exclusive distribution of the work in the form in which it was published by this journal, but provided that the link to the first publication of the article in this journal is preserved.
A license agreement is a document in which the author warrants that he/she owns all copyright for the work (manuscript, article, etc.).
The authors, signing the License Agreement with TECHNOLOGY CENTER PC, have all rights to the further use of their work, provided that they link to our edition in which the work was published.
According to the terms of the License Agreement, the Publisher TECHNOLOGY CENTER PC does not take away your copyrights and receives permission from the authors to use and dissemination of the publication through the world's scientific resources (own electronic resources, scientometric databases, repositories, libraries, etc.).
In the absence of a signed License Agreement or in the absence of this agreement of identifiers allowing to identify the identity of the author, the editors have no right to work with the manuscript.
It is important to remember that there is another type of agreement between authors and publishers – when copyright is transferred from the authors to the publisher. In this case, the authors lose ownership of their work and may not use it in any way.