Devising a deorbitation strategy for Kazakhstani’s KazEOSat-1 spacecraft
DOI:
https://doi.org/10.15587/1729-4061.2024.319226Keywords:
Spacecraft, deorbiting, satellite disposal, LEO, space debris, orbit, CubeSatAbstract
The object of this study is the process of deorbiting the KazEOSat-1 spacecraft, which has completed its active service life in low Earth orbit. The main problem is the lack of an effective technique to deorbit KazEOSat-1, taking into account its technical characteristics, orbital parameters, and the need to minimize risks to the environment and other objects in orbit.
As part of the work, a software model was built that takes into account the initial orbital parameters of the device, which are essential for planning and performing deorbiting maneuvers. The model is designed to accurately calculate the descent trajectory, taking into account the laws of celestial mechanics and the influence of atmospheric conditions. The optimal deorbiting strategy was selected based on an analysis of various methods for calculating orbital maneuvers aimed at reducing fuel consumption and minimizing environmental risks. This included a comparative analysis of existing approaches and the selection of the most suitable ones under the given mission parameters.
The results of the simulation using precise modeling methods in the MATLAB software environment allowed us to determine the main deorbiting parameters, such as the altitude at which the maneuvers begin, the required velocity impulses, the total fuel consumption, and the expected time before entering the dense layers of the atmosphere. Based on the obtained data, practical recommendations were formulated for the KazEOSat-1 deorbit. The first stage, the active controlled deorbit, is carried out by operating the low-thrust engine and braking by the Earth’s atmosphere, allowing the spacecraft to descend from 758 km to 444 km in 2.5 days. The second stage, the passive uncontrolled deorbit, continues the descent to 103 km in 969 days, using only atmospheric braking. The third stage, the uncontrolled drop, begins after reaching 103 km and ends with a drop to the Earth in 834 seconds
References
- Strategiya "Kazahstan-2050": noviy politicheskij kurs sostoyavshegosya gosudarstva. Informacionno-pravovaya sistema normativnyh pravovyh aktov Respubliki Kazahstan. Available at: https://adilet.zan.kz/rus/docs/K1200002050
- ISO 24113:2023. Space systems - Space debris mitigation requirements.
- Zhang, R., Yang, K., Zhang, J., Bi, S. (2024). Overview and Key Technology of the Membrane Drag Sail for Low Earth Orbit Satellite Deorbit. Space: Science & Technology, 4. https://doi.org/10.34133/space.0115
- Niccolai, L., Mengali, G. (2024). Performance Estimate of a Spin-Stabilized Drag Sail for Spacecraft Deorbiting. Applied Sciences, 14 (2), 612. https://doi.org/10.3390/app14020612
- Zhang, J., Zhang, R., Yang, K. (2022). Attitude Stability Analysis and Configuration Design of Pyramid Drag Sail for Deorbit Missions. Journal of Aerospace Engineering, 35 (6). https://doi.org/10.1061/(asce)as.1943-5525.0001479
- Xu, Y., Yang, Y., Huang, H., Jia, H., Fang, G. (2024). Numerical simulation of rigid-flexible coupled dynamics for an inflatable sphere deorbiting device. Advances in Space Research, 74 (1), 373–383. https://doi.org/10.1016/j.asr.2024.03.049
- Rioseco Olave, D., Caqueo Jara, N., Cassineli Palharini, R., Santos Araujo Palharini, R., Gaglio, E., Savino, R. (2023). Inflatable aerodynamic decelerator for CubeSat reentry and recovery: Altitude effects on the flowfield structure. Aerospace Science and Technology, 138, 108358. https://doi.org/10.1016/j.ast.2023.108358
- Caqueo, N., Palharini, R. C., Palharini, R. S. A., Gaglio, E., Savino, R. (2024). Inflatable aerodynamic decelerators for CubeSat reentry and recovery: Surface properties. Aerospace Science and Technology, 149, 109151. https://doi.org/10.1016/j.ast.2024.109151
- Xiao, H., Huang, J., Liu, G., Lv, Y. (2020). Space Environment Modeling and Deorbiting Efficiency Analysis for Electro-dynamic Tether System. 2020 Chinese Control And Decision Conference (CCDC), 5063–5067. https://doi.org/10.1109/ccdc49329.2020.9164373
- Sarego, G., Olivieri, L., Valmorbida, A., Brunello, A., Lorenzini, E. C., Tarabini Castellani, L. et al. (2021). Deployment requirements for deorbiting electrodynamic tether technology. CEAS Space Journal, 13 (4), 567–581. https://doi.org/10.1007/s12567-021-00349-5
- IADC Space Debris Mitigation Guidelines. Available at: https://orbitaldebris.jsc.nasa.gov/library/iadc-space-debris-guidelines-revision-2.pdf
- Vanessa, M. (Ed.) (2019). United Nations Handbook 2019–2020. Wellington: Ministry of Foreign Affairs and Trade of New Zealand, 58–60.
- Curtis, H. (2020). Orbital Mechanics for Engineering Students. Butterworth-Heinemann. https://doi.org/10.1016/c2016-0-02107-1
- Battin, R. H. (1999). An Introduction to the Mathematics and Methods of Astrodynamics, Revised Edition. AIAA. https://doi.org/10.2514/4.861543
- Brophy, J. R. (2000). Low-Thrust Propulsion Technology. Journal of Propulsion and Power.
- Janovsky, R., Kassebom, M., Lübberstedt, H., Romberg, O. et al. (2003). End-of-Life de -Orbiting Strategies for Satellites. 54th International Astronautical Congress of the International Astronautical Federation, the International Academy of Astronautics, and the International Institute of Space Law. https://doi.org/10.2514/6.iac-03-iaa.5.4.05
- Everetts, W., Rock, K., Iovanov, M. (2020). Iridium deorbit strategy, execution, and results. Journal of Space Safety Engineering, 7 (3), 351–357. https://doi.org/10.1016/j.jsse.2020.07.013
- NASA Orbital Debris Program Office. Available at: https://orbitaldebris.jsc.nasa.gov/
- Space Debris. Available at: https://www.esa.int/Space_Safety/Space_Debris
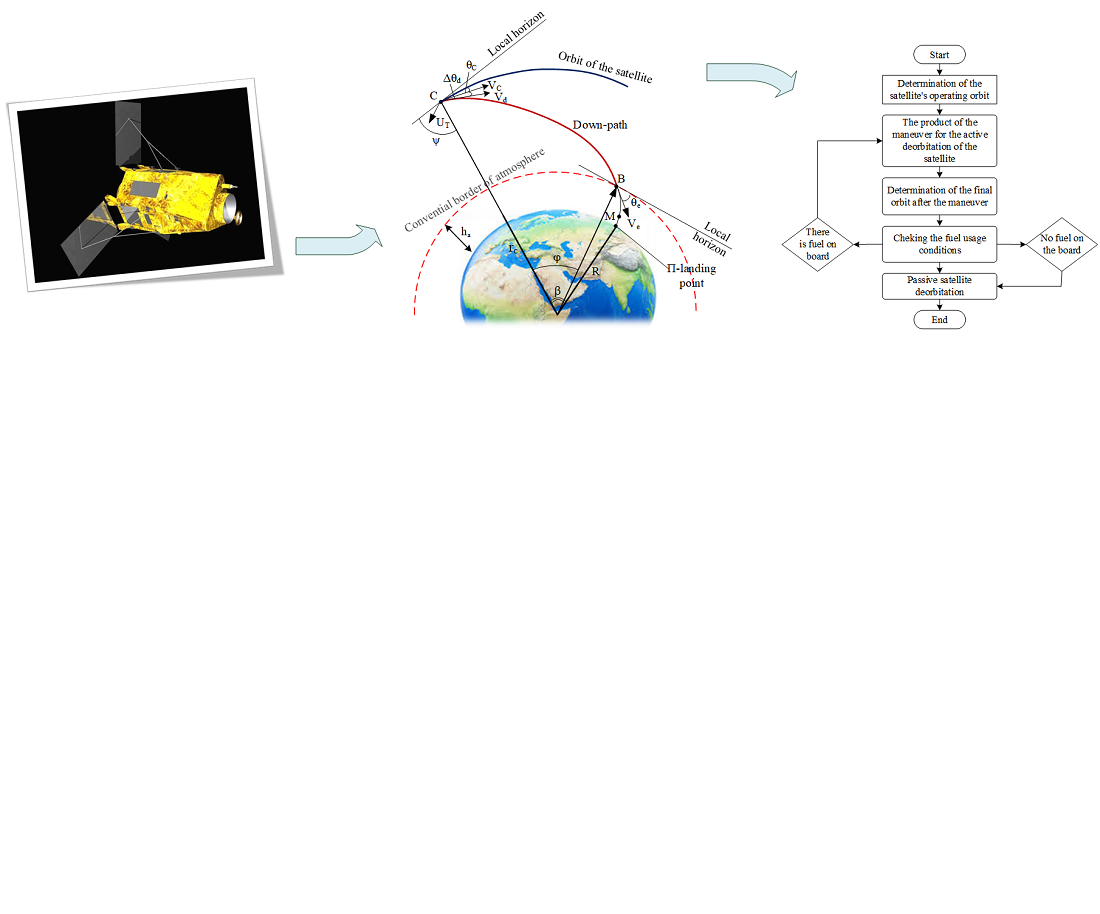
Downloads
Published
How to Cite
Issue
Section
License
Copyright (c) 2024 Berik Zhumazhanov, Aigul Kulakayeva, Abdikul Ashurov, Kazbek Baktybekov, Ainur Zhetpisbayeva, Daniyar Uskenbaev, Bexultan Zhumazhanov, Aigerim Zylgara, Aliya Kargulova

This work is licensed under a Creative Commons Attribution 4.0 International License.
The consolidation and conditions for the transfer of copyright (identification of authorship) is carried out in the License Agreement. In particular, the authors reserve the right to the authorship of their manuscript and transfer the first publication of this work to the journal under the terms of the Creative Commons CC BY license. At the same time, they have the right to conclude on their own additional agreements concerning the non-exclusive distribution of the work in the form in which it was published by this journal, but provided that the link to the first publication of the article in this journal is preserved.
A license agreement is a document in which the author warrants that he/she owns all copyright for the work (manuscript, article, etc.).
The authors, signing the License Agreement with TECHNOLOGY CENTER PC, have all rights to the further use of their work, provided that they link to our edition in which the work was published.
According to the terms of the License Agreement, the Publisher TECHNOLOGY CENTER PC does not take away your copyrights and receives permission from the authors to use and dissemination of the publication through the world's scientific resources (own electronic resources, scientometric databases, repositories, libraries, etc.).
In the absence of a signed License Agreement or in the absence of this agreement of identifiers allowing to identify the identity of the author, the editors have no right to work with the manuscript.
It is important to remember that there is another type of agreement between authors and publishers – when copyright is transferred from the authors to the publisher. In this case, the authors lose ownership of their work and may not use it in any way.