Increasing the reliability of biomass solid fuel combustion using a combined regenerative heat exchanger as an indirect burner
DOI:
https://doi.org/10.15587/1729-4061.2022.265803Keywords:
biomass, indirect burner, regenerative heat exchanger, regenerator, mantle heat exchangerAbstract
In this study, an indirect burner system for solid biomass fuel is designed. The design is motivated by the need to solve the problem related to a direct burner system, such as slagging and high pollutant emissions due to the high-temperature burning process. Therefore, the utilization of an indirect burner is expected to improve the reliability of the solid biomass combustion process. It also can be used to reduce coal consumption by using an indirect burner where the working fluid reaches a relatively higher temperature before entering the boiler. The design used the first principle method for creating the regenerator heat exchanger. The regenerator consists of a mantle and coil heat exchanger. The test used solid biomass fuel for the combustion process where the working fluid first enters the mantle heat exchanger and then the coil heat exchanger. As a result, the mantle absorbs sufficient heat losses from the combustion chamber with the highest temperature increment of 19 °C. The warm water from the mantle then flows to the coil arrangement within the combustion chamber. As a result, the highest temperature of the coil is 84.5 °C. The heat transfer rate for the coil and mantle is 57.2–85.6 and 124.9–141.5 W. The key finding is that the combined regenerative heat exchanger can deliver a higher transfer rate. This can be achieved since the heat exchanger utilizes the same flow distribution, increasing the mean temperature differences at the inlet. Thus, it can produce an average heat transfer rate of 210.5 W. Therefore, energy consumption for coal or other fossil fuels can be reduced significantly. The data can be used for further improvement of the existing boiler system and help to increase the thermal efficiency of the system.
References
- Ismail, I., Pane, E. A., Haryanto, G., Okviyanto, T., Rahman, R. A. (2021). A Better Approach for Modified Bach-Type Savonius Turbine Optimization. International Review of Aerospace Engineering (IREASE), 14 (3), 159–165. doi: https://doi.org/10.15866/irease.v14i3.20612
- Deng, J., Furbo, S., Kong, W., Fan, J. (2018). Thermal performance assessment and improvement of a solar domestic hot water tank with PCM in the mantle. Energy and Buildings, 172, 10–21. doi: https://doi.org/10.1016/j.enbuild.2018.04.058
- Saukenova, I., Oliskevych, M., Taran, I., Toktamyssova, A., Aliakbarkyzy, D., Pelo, R. (2022). Optimization of schedules for early garbage collection and disposal in the megapolis. Eastern-European Journal of Enterprise Technologies, 1 (3 (115)), 13–23. doi: https://doi.org/10.15587/1729-4061.2022.251082
- Wiyono, A., Saw, L. H., Anggrainy, R., Husen, A. S., Purnawan, Rohendi, D., Gandidi, I. M., Adanta, D., Pambudi, N. A. (2021). Enhancement of syngas production via co-gasification and renewable densified fuels (RDF) in an open-top downdraft gasifier: Case study of Indonesian waste. Case Studies in Thermal Engineering, 27, 101205. doi: https://doi.org/10.1016/j.csite.2021.101205
- Gandidi, I. M., Wiyono, A., Berman, E. T., Pambudi, N. A. (2019). Experimental upgrading of liquid crude oil obtained from calophyllum inophyllum by two-stage pyrolysis. Case Studies in Thermal Engineering, 16, 100544. doi: https://doi.org/10.1016/j.csite.2019.100544
- Adanta, D., Syofii, I., Sari, D., Wiyono, A. (2022). Performance of Pico Scale Turgo Turbine in Difference the Nozzle Diameter. International Journal of Fluid Machinery and Systems, 15 (1), 130–136. doi: https://doi.org/10.5293/ijfms.2022.15.1.130
- Rahmalina, D., Adhitya, D. C., Rahman, R. A., Ismail, I. (2021). Improvement the performance of composite PCM paraffin-based incorporate with volcanic ash as heat storage for low-temperature application. EUREKA: Physics and Engineering, 1, 53–61. doi: https://doi.org/10.21303/2461-4262.2022.002055
- Ismail, I., Mulyanto, A. T., Rahman, R. A. (2022). Development of free water knock-out tank by using internal heat exchanger for heavy crude oil. EUREKA: Physics and Engineering, 4, 77–85. doi: https://doi.org/10.21303/2461-4262.2022.002502
- Kumar, A., Bhattacharya, T., Mozammil Hasnain, S. M., Kumar Nayak, A., Hasnain, M. S. (2020). Applications of biomass-derived materials for energy production, conversion, and storage. Materials Science for Energy Technologies, 3, 905–920. doi: https://doi.org/10.1016/j.mset.2020.10.012
- Tarigan, E. (2018). Mathematical modeling and simulation of a solar agricultural dryer with back-up biomass burner and thermal storage. Case Studies in Thermal Engineering, 12, 149–165. doi: https://doi.org/10.1016/j.csite.2018.04.012
- Hroncová, E., Ladomersky, J., Valíček, J., Dzurenda, L. (2016). Combustion of Biomass Fuel and Residues: Emissions Production Perspective. Developments in Combustion Technology, 3–32. doi: https://doi.org/10.5772/63793
- Koyfman, O., Simkin, A., Kravchenko, V., Vorotnikova, Z. (2022). Development of a mathematical model to monitoring the velocity of subsidence of charge material column in the blast furnace based on the parameters of gas pressure in the furnace tract. Eastern-European Journal of Enterprise Technologies, 1 (2 (115)), 116–126. doi: https://doi.org/10.15587/1729-4061.2022.246175
- Novikov, P., Shtifzon, O., Bunke, O., Batiuk, S. (2022). Selecting a method for the parametric adaptation of pi-controller in the control systems of boiler assemblies at thermal power stations with supercritical parameters. Eastern-European Journal of Enterprise Technologies, 2 (2 (116)), 61–68. doi: https://doi.org/10.15587/1729-4061.2022.254116
- Rahmalina, D., Rahman, R. A., Ismail. (2022). Increasing the rating performance of paraffin up to 5000 cycles for active latent heat storage by adding high-density polyethylene to form shape-stabilized phase change material. Journal of Energy Storage, 46, 103762. doi: https://doi.org/10.1016/j.est.2021.103762
- Mousavi, S. M., Fatehi, H., Bai, X.-S. (2021). Numerical study of the combustion and application of SNCR for NO reduction in a lab-scale biomass boiler. Fuel, 293, 120154. doi: https://doi.org/10.1016/j.fuel.2021.120154
- Mlonka-Mędrala, A., Dziok, T., Magdziarz, A., Nowak, W. (2021). Composition and properties of fly ash collected from a multifuel fluidized bed boiler co-firing refuse derived fuel (RDF) and hard coal. Energy, 234, 121229. doi: https://doi.org/10.1016/j.energy.2021.121229
- Aranguren, M., Castillo-Villar, K. K., Aboytes-Ojeda, M. (2021). A two-stage stochastic model for co-firing biomass supply chain networks. Journal of Cleaner Production, 319, 128582. doi: https://doi.org/10.1016/j.jclepro.2021.128582
- Drosatos, P., Nikolopoulos, N., Karampinis, E., Strotos, G., Grammelis, P., Kakaras, E. (2020). Numerical comparative investigation of a flexible lignite-fired boiler using pre-dried lignite or biomass as supporting fuel. Renewable Energy, 145, 1831–1848. doi: https://doi.org/10.1016/j.renene.2019.07.071
- Chen, C., Bi, Y., Huang, Y., Huang, H. (2021). Review on slagging evaluation methods of biomass fuel combustion. Journal of Analytical and Applied Pyrolysis, 155, 105082. doi: https://doi.org/10.1016/j.jaap.2021.105082
- Rahman, R. A., Suwandi, A., Nurtanto, M. (2021). Experimental investigation on the effect of thermophysical properties of a heat transfer fluid on pumping performance for a convective heat transfer system. Journal of Thermal Engineering, 7 (7), 1628–1639. doi: https://doi.org/10.18186/thermal.1025910
- Torelló, À., Defay, E. (2021). Heat exchange law in caloric regenerators. International Journal of Refrigeration, 127, 174–179. doi: https://doi.org/10.1016/j.ijrefrig.2021.02.024
- Qian, S., Yu, J., Yan, G. (2017). A review of regenerative heat exchange methods for various cooling technologies. Renewable and Sustainable Energy Reviews, 69, 535–550. doi: https://doi.org/10.1016/j.rser.2016.11.180
- Aviso, K. B., Sy, C. L., Tan, R. R., Ubando, A. T. (2020). Fuzzy optimization of carbon management networks based on direct and indirect biomass co-firing. Renewable and Sustainable Energy Reviews, 132, 110035. doi: https://doi.org/10.1016/j.rser.2020.110035
- Yang, B., Wei, Y.-M., Liu, L.-C., Hou, Y.-B., Zhang, K., Yang, L., Feng, Y. (2021). Life cycle cost assessment of biomass co-firing power plants with CO2 capture and storage considering multiple incentives. Energy Economics, 96, 105173. doi: https://doi.org/10.1016/j.eneco.2021.105173
- Milićević, A., Belošević, S., Crnomarković, N., Tomanović, I., Stojanović, A., Tucaković, D. et. al. (2021). Numerical study of co-firing lignite and agricultural biomass in utility boiler under variable operation conditions. International Journal of Heat and Mass Transfer, 181, 121728. doi: https://doi.org/10.1016/j.ijheatmasstransfer.2021.121728
- Tsekos, C., del Grosso, M., de Jong, W. (2021). Gasification of woody biomass in a novel indirectly heated bubbling fluidized bed steam reformer. Fuel Processing Technology, 224, 107003. doi: https://doi.org/10.1016/j.fuproc.2021.107003
- Ghiami, S., Khallaghi, N., Borhani, T. N. (2021). Techno-economic and environmental assessment of staged oxy-co-firing of biomass-derived syngas and natural gas. Energy Conversion and Management, 243, 114410. doi: https://doi.org/10.1016/j.enconman.2021.114410
- Smoliński, A., Howaniec, N., Gąsior, R., Polański, J., Magdziarczyk, M. (2021). Hydrogen rich gas production through co-gasification of low rank coal, flotation concentrates and municipal refuse derived fuel. Energy, 235, 121348. doi: https://doi.org/10.1016/j.energy.2021.121348
- Ndukwu, M. C., Diemuodeke, E. O., Abam, F. I., Abada, U. C., Eke-emezie, N., Simo-Tagne, M. (2020). Development and modelling of heat and mass transfer analysis of a low-cost solar dryer integrated with biomass heater: Application for West African Region. Scientific African, 10, e00615. doi: https://doi.org/10.1016/j.sciaf.2020.e00615
- Lazova, M., Huisseune, H., Kaya, A., Lecompte, S., Kosmadakis, G., De Paepe, M. (2016). Performance Evaluation of a Helical Coil Heat Exchanger Working under Supercritical Conditions in a Solar Organic Rankine Cycle Installation. Energies, 9 (6), 432. doi: https://doi.org/10.3390/en9060432
- Chikhi, M., Sellami, R., Merzouk, N. K. (2014). Thermal Properties Study of a Solar Water Heater Tank with a Mantle Exchanger. International Journal of Energy Optimization and Engineering, 3 (1), 92–100. doi: https://doi.org/10.4018/ijeoe.2014010106
- Jaluria, Y. (2016). Design and Optimization of Thermal Systems (Vol. 4, Issue 2). Taylor & Francis Group.
- Kilkovský, B. (2020). Review of Design and Modeling of Regenerative Heat Exchangers. Energies, 13 (3), 759. doi: https://doi.org/10.3390/en13030759
- Gao, J., Tian, G., Sorniotti, A., Karci, A. E., Di Palo, R. (2019). Review of thermal management of catalytic converters to decrease engine emissions during cold start and warm up. Applied Thermal Engineering, 147, 177–187. doi: https://doi.org/10.1016/j.applthermaleng.2018.10.037
- Arslan, M., Igci, A. A. (2015). Thermal performance of a vertical solar hot water storage tank with a mantle heat exchanger depending on the discharging operation parameters. Solar Energy, 116, 184–204. doi: https://doi.org/10.1016/j.solener.2015.03.045
- Afsharpanah, F., Mousavi Ajarostaghi, S. S., Sedighi, K. (2019). The influence of geometrical parameters on the ice formation enhancement in a shell and double coil ice storage system. SN Applied Sciences, 1 (10). doi: https://doi.org/10.1007/s42452-019-1317-3
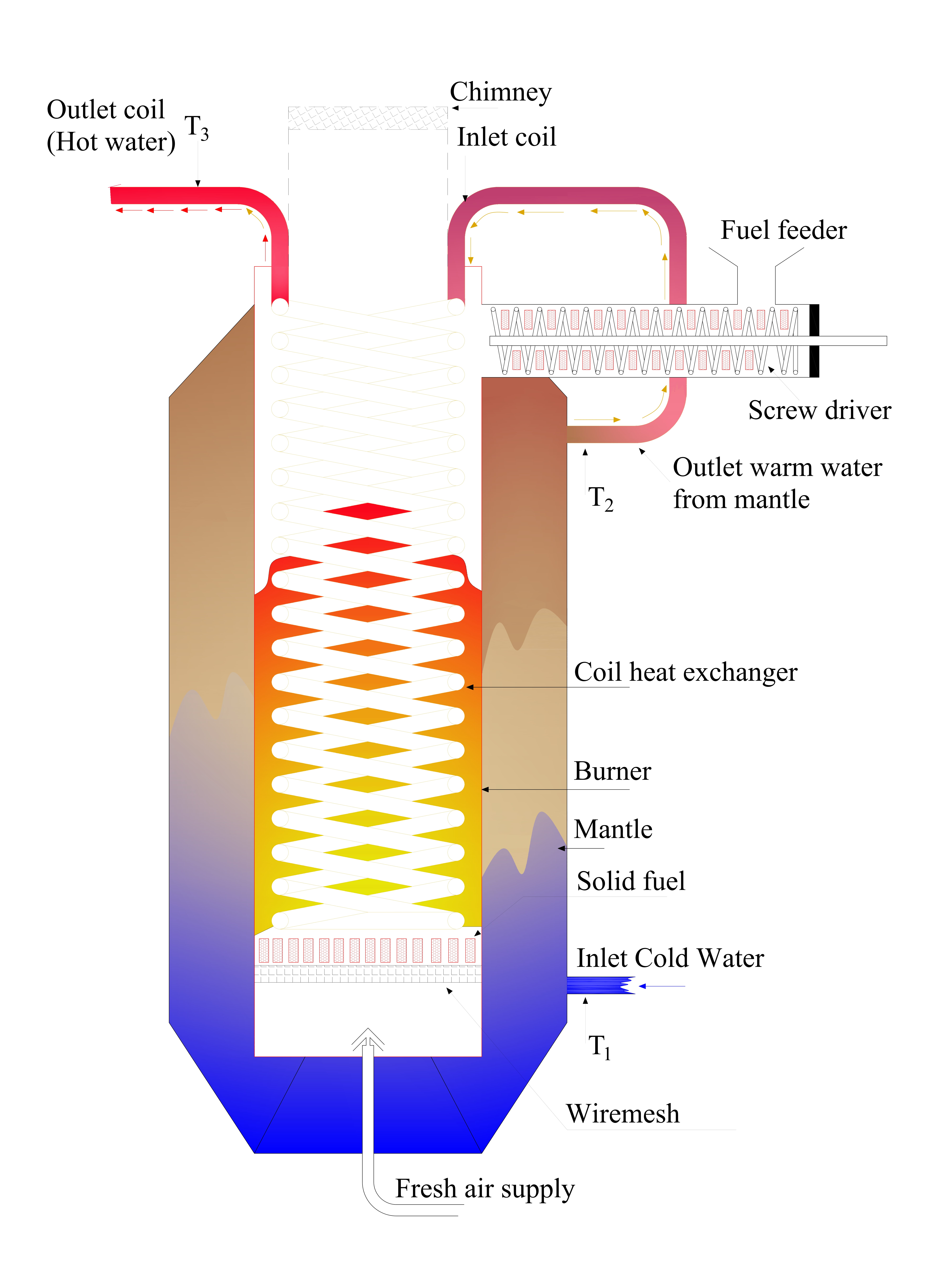
Downloads
Published
How to Cite
Issue
Section
License
Copyright (c) 2022 Alwinsyah Tunggul Ismail, Ismail Ismail, Reza Abdu Rahman

This work is licensed under a Creative Commons Attribution 4.0 International License.
The consolidation and conditions for the transfer of copyright (identification of authorship) is carried out in the License Agreement. In particular, the authors reserve the right to the authorship of their manuscript and transfer the first publication of this work to the journal under the terms of the Creative Commons CC BY license. At the same time, they have the right to conclude on their own additional agreements concerning the non-exclusive distribution of the work in the form in which it was published by this journal, but provided that the link to the first publication of the article in this journal is preserved.
A license agreement is a document in which the author warrants that he/she owns all copyright for the work (manuscript, article, etc.).
The authors, signing the License Agreement with TECHNOLOGY CENTER PC, have all rights to the further use of their work, provided that they link to our edition in which the work was published.
According to the terms of the License Agreement, the Publisher TECHNOLOGY CENTER PC does not take away your copyrights and receives permission from the authors to use and dissemination of the publication through the world's scientific resources (own electronic resources, scientometric databases, repositories, libraries, etc.).
In the absence of a signed License Agreement or in the absence of this agreement of identifiers allowing to identify the identity of the author, the editors have no right to work with the manuscript.
It is important to remember that there is another type of agreement between authors and publishers – when copyright is transferred from the authors to the publisher. In this case, the authors lose ownership of their work and may not use it in any way.