Assessing the effect of mechanical deformation of the panasonic NCR18650B lithium-ion power cell housing on its fire safety
DOI:
https://doi.org/10.15587/1729-4061.2023.276780Keywords:
Panasonic NCR18650B, mechanical deformation, combustion temperature, mathematical model, fire hazardAbstract
This paper considers the deformation properties of the body of the lithium-ion power cell (LIPC) Panasonic NCR18650B (LiNi0.8Co0.15Al0.05O2) exposed to the action of static load at various techniques of fixing the cell. Determining the properties of LIPCs under appropriate conditions makes it possible to fill the gap in existing studies, which will further ensure the safety of their use.
Based on the results of experimental studies, the LIPC rigidity and temperature indicators were determined in accordance with the applied load. The most dangerous variant, from the point of view of fire danger, of applying a static load on the cell has been established.
It was experimentally established that, on average, the Panasonic NCR18650B LIPC housing can withstand a load of about 80 kg·s/cm2 (or 7.84 MPa) without further ignition. An increase in pressure force in the range exceeding 85–90 kg·s/cm2 leads to an irreversible chain thermochemical reaction, which, within 2–3 seconds, leads to LIPC ignition. Compressing the LIPC evenly along its lateral surface showed the occurrence of combustion at the load on the cell equal to 150 kg·s/cm2. The average temperature of the cell during combustion caused by the deformation of the housing is 350–450 °C, and the maximum value is registered in the range of 580–680 °C.
The mathematical model built on the basis of the mathematical theory of thin shells adequately describes the stressed-strained state of the cylindrical body of cells under the action of a force concentrated and distributed load. The estimation model is satisfactorily verified by experimental results, making it possible to improve the strength and rigidity of LIPC housing by choosing the appropriate steel grade for its body, the geometric dimensions, and the structural technique of its fastening.
References
- Cai, Y., Ku, L., Wang, L., Ma, Y., Zheng, H., Xu, W. et al. (2019). Engineering oxygen vacancies in hierarchically Li-rich layered oxide porous microspheres for high-rate lithium ion battery cathode. Science China Materials, 62 (10), 1374–1384. doi: https://doi.org/10.1007/s40843-019-9456-1
- Chen, X., Li, H., Yan, Z., Cheng, F., Chen, J. (2019). Structure design and mechanism analysis of silicon anode for lithium-ion batteries. Science China Materials, 62 (11), 1515–1536. doi: https://doi.org/10.1007/s40843-019-9464-0
- Lazarenko, O., Berezhanskyi, T., Pospolitak, V., Pazen, O. (2022). Experimental evaluation of the influence of excessive electric current on the fire hazard of lithium-ion power cell. Eastern-European Journal of Enterprise Technologies, 4 (10 (118)), 67–75. doi: https://doi.org/10.15587/1729-4061.2022.263001
- Lazarenko, O. V., Pazen, O. Y., Sukach, R. Y., Pospolitak, V. I. (2022). Experimental evaluation of fire hazard of lithium-ion battery during its mechanical damage. Naukovyi Visnyk Natsionalnoho Hirnychoho Universytetu, 5, 68–73. doi: https://doi.org/10.33271/nvngu/2022-5/068
- Chen, Y., Kang, Y., Zhao, Y., Wang, L., Liu, J., Li, Y. et al. (2021). A review of lithium-ion battery safety concerns: The issues, strategies, and testing standards. Journal of Energy Chemistry, 59, 83–99. doi: https://doi.org/10.1016/j.jechem.2020.10.017
- GB/T 31485-2015. Safety requirements and test methods for traction battery of electric vehicle (English Version). Available at: https://www.codeofchina.com/standard/GBT31485-2015.html
- Ruiz, V., Pfrang, A., Kriston, A., Omar, N., Van den Bossche, P., Boon-Brett, L. (2018). A review of international abuse testing standards and regulations for lithium ion batteries in electric and hybrid electric vehicles. Renewable and Sustainable Energy Reviews, 81, 1427–1452. doi: https://doi.org/10.1016/j.rser.2017.05.195
- Xi, S., Chang, L., Chen, W., Zhao, Q., Guo, Y., Cai, Z. (2021). Mechanical Response Analysis of Battery Modules Under Mechanical Load: Experimental Investigation and Simulation Analysis. Energy Technology, 10 (3), 2100763. doi: https://doi.org/10.1002/ente.202100763
- Kermani, G., Keshavarzi, M. M., Sahraei, E. (2021). Deformation of lithium-ion batteries under axial loading: Analytical model and Representative Volume Element. Energy Reports, 7, 2849–2861. doi: https://doi.org/10.1016/j.egyr.2021.05.015
- Yuan, Q., Chen, X., Meng, K., Wang, P., Tang, L., Wang, T. et al. (2022). Research on Mechanical Simulation Model and Working Safety Boundary of Large-Capacity Prismatic Lithium-Ion Battery Based on Experiment. Journal of Electrochemical Energy Conversion and Storage, 19 (3). doi: https://doi.org/10.1115/1.4054062
- Wang, L., Yin, S., Yu, Z., Wang, Y., Yu, T. X., Zhao, J. et al. (2018). Unlocking the significant role of shell material for lithium-ion battery safety. Materials & Design, 160, 601–610. doi: https://doi.org/10.1016/j.matdes.2018.10.002
- Xi, S., Zhao, Q., Chang, L., Huang, X., Cai, Z. (2020). The dynamic failure mechanism of a lithium-ion battery at different impact velocity. Engineering Failure Analysis, 116, 104747. doi: https://doi.org/10.1016/j.engfailanal.2020.104747
- Xing, B., Xiao, F., Korogi, Y., Ishimaru, T., Xia, Y. (2021). Direction-dependent mechanical-electrical-thermal responses of large-format prismatic Li-ion battery under mechanical abuse. Journal of Energy Storage, 43, 103270. doi: https://doi.org/10.1016/j.est.2021.103270
- Perea, A., Paolella, A., Dubé, J., Champagne, D., Mauger, A., Zaghib, K. (2018). State of charge influence on thermal reactions and abuse tests in commercial lithium-ion cells. Journal of Power Sources, 399, 392–397. doi: https://doi.org/10.1016/j.jpowsour.2018.07.112
- Muresanu, A. D., Dudescu, M. C. (2022). Numerical and Experimental Evaluation of a Battery Cell under Impact Load. Batteries, 8 (5), 48. doi: https://doi.org/10.3390/batteries8050048
- Yin, H., Ma, S., Li, H., Wen, G., Santhanagopalan, S., Zhang, C. (2021). Modeling strategy for progressive failure prediction in lithium-ion batteries under mechanical abuse. ETransportation, 7, 100098. doi: https://doi.org/10.1016/j.etran.2020.100098
- Lazarenko, O., Pospolitak, V. (2021). Methods of testing lithium-ion batteries for fire hazard. Fire Safety, 39, 49–55. doi: https://doi.org/10.32447/20786662.39.2021.06
- Sheikh, M., Elmarakbi, M., Rehman, S., Elmarakbi, A. (2021). Internal Short Circuit Analysis of Cylindrical Lithium-Ion Cells Due to Structural Failure. Journal of The Electrochemical Society, 168(3), 030526. doi: https://doi.org/10.1149/1945-7111/abec54
- Zhang, X., Wierzbicki, T. (2015). Characterization of plasticity and fracture of shell casing of lithium-ion cylindrical battery. Journal of Power Sources, 280, 47–56. doi: https://doi.org/10.1016/j.jpowsour.2015.01.077
- Material No.: AISI 321. Available at: https://woite-edelstahl.com/aisi321en.html
- Xia, Y., Wierzbicki, T., Sahraei, E., Zhang, X. (2014). Damage of cells and battery packs due to ground impact. Journal of Power Sources, 267, 78–97. doi: https://doi.org/10.1016/j.jpowsour.2014.05.078
- Kisters, T., Sahraei, E., Wierzbicki, T. (2017). Dynamic impact tests on lithium-ion cells. International Journal of Impact Engineering, 108, 205–216. doi: https://doi.org/10.1016/j.ijimpeng.2017.04.025
- Xu, J., Liu, B., Wang, L., Shang, S. (2015). Dynamic mechanical integrity of cylindrical lithium-ion battery cell upon crushing. Engineering Failure Analysis, 53, 97–110. doi: https://doi.org/10.1016/j.engfailanal.2015.03.025
- Xu, J., Liu, B., Wang, X., Hu, D. (2016). Computational model of 18650 lithium-ion battery with coupled strain rate and SOC dependencies. Applied Energy, 172, 180–189. doi: https://doi.org/10.1016/j.apenergy.2016.03.108
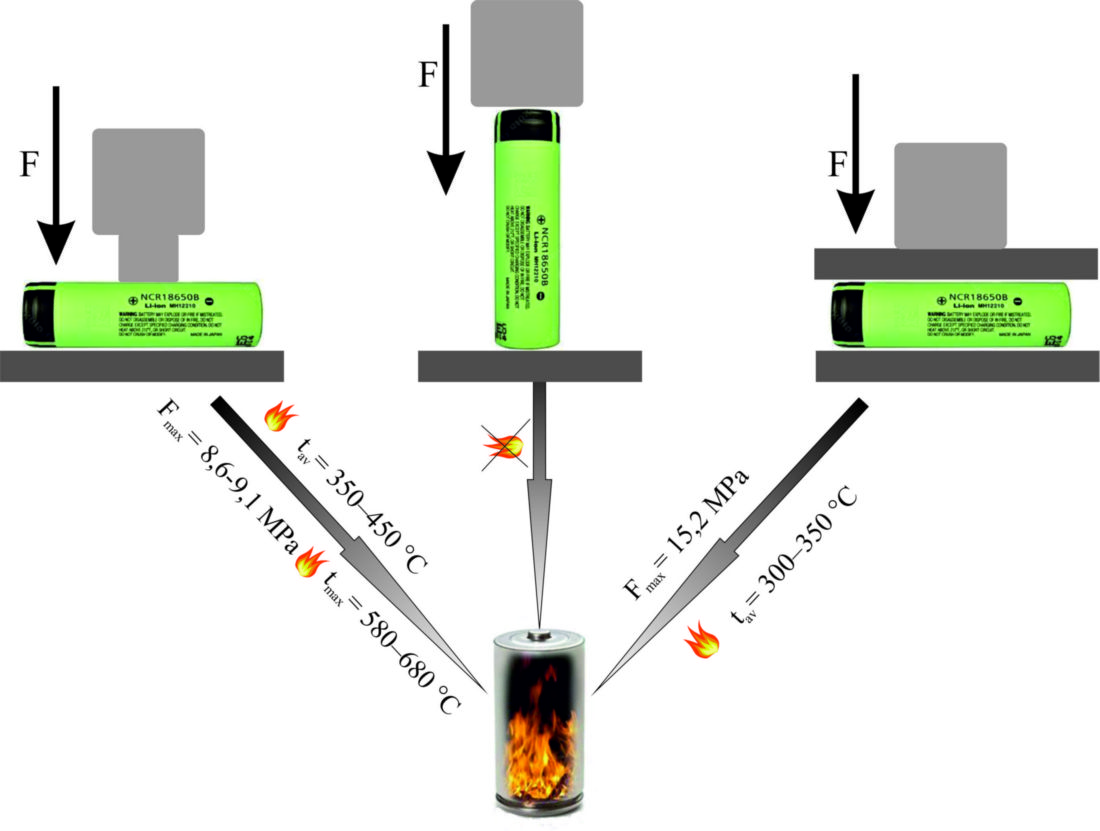
Downloads
Published
How to Cite
Issue
Section
License
Copyright (c) 2023 Oleksandr Lazarenko, Taras Hembara, Vitalii Pospolitak, Dmytro Voytovych

This work is licensed under a Creative Commons Attribution 4.0 International License.
The consolidation and conditions for the transfer of copyright (identification of authorship) is carried out in the License Agreement. In particular, the authors reserve the right to the authorship of their manuscript and transfer the first publication of this work to the journal under the terms of the Creative Commons CC BY license. At the same time, they have the right to conclude on their own additional agreements concerning the non-exclusive distribution of the work in the form in which it was published by this journal, but provided that the link to the first publication of the article in this journal is preserved.
A license agreement is a document in which the author warrants that he/she owns all copyright for the work (manuscript, article, etc.).
The authors, signing the License Agreement with TECHNOLOGY CENTER PC, have all rights to the further use of their work, provided that they link to our edition in which the work was published.
According to the terms of the License Agreement, the Publisher TECHNOLOGY CENTER PC does not take away your copyrights and receives permission from the authors to use and dissemination of the publication through the world's scientific resources (own electronic resources, scientometric databases, repositories, libraries, etc.).
In the absence of a signed License Agreement or in the absence of this agreement of identifiers allowing to identify the identity of the author, the editors have no right to work with the manuscript.
It is important to remember that there is another type of agreement between authors and publishers – when copyright is transferred from the authors to the publisher. In this case, the authors lose ownership of their work and may not use it in any way.