Estimation of electron density, temperature and electrical characterization of silica seeded arc plasma at atmospheric pressure
DOI:
https://doi.org/10.15587/1729-4061.2023.289006Keywords:
arc discharge, silica seeding, electron density, electron temperature, Langmuir probesAbstract
Plasma technology stands at the forefront of numerous industrial applications, offering versatile solutions from materials processing to aerospace engineering. This study employs a single Langmuir probe technique operating at atmospheric pressure to scrutinize the transformative impact of silica seeding on low-temperature arc plasma. The investigation unveils a dynamic interplay of electrons and ions within the plasma, unveiling key electrical properties. The I–V electrical properties of the arcs plasma before seeding, having a floating voltage of –39 V, demonstrate electron and ion currents for varied probe voltages. The electrons’ density is calculated to be 2.11×1013 m–3, and the electrons’ temperature is at 6.25 eV. The I–V characteristics show a floating potential of about –35 V and –37 V after seeding an arc plasma using silica in the presence of aluminum oxide (2 % by weight) powder and grain, respectively. After seeding, it is discovered that the electron temperature falls to 1.18 eV for powder while 1.16 eV for grain and electron density rises to 2×1016 m–3 for powder and 1.84×1016 m–3 for grain. In addition, a notable fall in electron temperature and a discernible rise in electron density are seen. This non-equilibrium behavior is related to silica’s catalytic function, which is enhanced by the presence of aluminum oxide. Additionally, increased ionizing activity brought on by inelastic electron collisions causes the electron temperatures in the silica-seeded arcs plasma to rise with discharge voltage. These findings can be essential for enhancing plasma-based technologies in a variety of industrial applications because they provide insightful information on how silica seeding affects arc plasma properties
Supporting Agency
- Financial support of the University Grants Commission (UGC), Nepal is very much appreciated.
References
- Shah, A. K., Shrestha, R., Sah, R. L., Nakarmi, J. J., Mishra, L. N. (2022). Experimental study of dielectric barrier discharge in an atmospheric air pressure and its electrical characterization. JP Journal of Heat and Mass Transfer, 30, 135–150. doi: https://doi.org/10.17654/0973576322060
- Dolai, B., Prajapati, R. P. (2017). Rayleigh-Taylor instability and internal waves in strongly coupled quantum plasma. Physics of Plasmas, 24 (11). doi: https://doi.org/10.1063/1.5000414
- Thakur, G., Khanal, R., Narayan, B. (2019). Characterization of Arc Plasma by Movable Single and Double Langmuir Probes. Fusion Science and Technology, 75 (4), 324–329. doi: https://doi.org/10.1080/15361055.2019.1579623
- Mishra, L. N., Shibata, K., Ito, H., Yugami, N., Nishida, Y. (2003). Characteristics of electron cyclotron resonance plasma generated in a rectangular waveguide by high-power microwave. Review of Scientific Instruments, 75 (1), 84–89. doi: https://doi.org/10.1063/1.1630858
- Brockhaus, A., Borchardt, C., Engemann, J. (1994). Langmuir probe measurements in commercial plasma plants. Plasma Sources Science and Technology, 3 (4), 539–544. doi: https://doi.org/10.1088/0963-0252/3/4/011
- Ben Salem, D., Carton, O., Fakhouri, H., Pulpytel, J., Arefi-Khonsari, F. (2014). Deposition of Water Stable Plasma Polymerized Acrylic Acid/MBA Organic Coatings by Atmospheric Pressure Air Plasma Jet. Plasma Processes and Polymers, 11 (3), 269–278. doi: https://doi.org/10.1002/ppap.201300064
- Shakya, A., Baniya, H. B., Pradhan, S. P., Basnet, N., Adhikari, R., Subedi, D. P., Regmi, S. (2022). Cold Plasma as a Practical Approach to Cancer Treatment. Plasma Medicine, 12 (4), 57–73. doi: https://doi.org/10.1615/plasmamed.2023047628
- Olszewski, P., Li, J. F., Liu, D. X., Walsh, J. L. (2014). Optimizing the electrical excitation of an atmospheric pressure plasma advanced oxidation process. Journal of Hazardous Materials, 279, 60–66. doi: https://doi.org/10.1016/j.jhazmat.2014.06.059
- Mizuno, A., Yamazaki, Y., Ito, H., Yoshida, H. (1992). AC energized ferroelectric pellet bed gas cleaner. IEEE Transactions on Industry Applications, 28 (3), 535–540. doi: https://doi.org/10.1109/28.137431
- Francke, K.-P., Miessner, H., Rudolph, R. (2000). Plasmacatalytic processes for environmental problems. Catalysis Today, 59 (3-4), 411–416. doi: https://doi.org/10.1016/s0920-5861(00)00306-0
- Boutonnet Kizling, M., Järås, S. G. (1996). A review of the use of plasma techniques in catalyst preparation and catalytic reactions. Applied Catalysis A: General, 147 (1), 1–21. doi: https://doi.org/10.1016/s0926-860x(96)00215-3
- Bromberg, L., Cohn, D. R., Rabinovich, A., O’Brie, C., Hochgreb, S. (1998). Plasma Reforming of Methane. Energy & Fuels, 12 (1), 11–18. doi: https://doi.org/10.1021/ef9701091
- Korzhyk, V., Khaskin, V., Grynyuk, A., Ganushchak, O., Peleshenko, S., Konoreva, O. et al. (2021). Comparing features in metallurgical interaction when applying different techniques of arc and plasma surfacing of steel wire on titanium. Eastern-European Journal of Enterprise Technologies, 4 (12 (112)), 6–17. doi: https://doi.org/10.15587/1729-4061.2021.238634
- Schmidt-Szałowski, K., Borucka, A., Jodzis, S. (1990). Catalytic activity of silica in ozone formation in electrical discharges. Plasma Chemistry and Plasma Processing, 10 (3), 443–450. doi: https://doi.org/10.1007/bf01447202
- Gruenwald, J., Reynvaan, J., Geistlinger, P. (2018). Basic plasma parameters and physical properties of inverted He fireballs. Plasma Sources Science and Technology, 27 (1), 015008. doi: https://doi.org/10.1088/1361-6595/aaa332
- Nagi, Ł., Kozioł, M., Zygarlicki, J. (2020). Comparative Analysis of Optical Radiation Emitted by Electric Arc Generated at AC and DC Voltage. Energies, 13 (19), 5137. doi: https://doi.org/10.3390/en13195137
- Armijo, K., Clem, P., Kotovsky, D., Demosthenous, B., Tanbakuchi, A., Martinez, R., Muna, A., LaFleur, C. (2019). Electrical Arc Fault Particle Size Characterization. doi: https://doi.org/10.2172/1592574
- Shigeta, M., Hirayama, Y., Ghedini, E. (2021). Computational Study of Quenching Effects on Growth Processes and Size Distributions of Silicon Nanoparticles at a Thermal Plasma Tail. Nanomaterials, 11 (6), 1370. doi: https://doi.org/10.3390/nano11061370
- Shigeta, M., Tanaka, M., Ghedini, E. (2019). Numerical Analysis of the Correlation between Arc Plasma Fluctuation and Nanoparticle Growth–Transport under Atmospheric Pressure. Nanomaterials, 9 (12), 1736. doi: https://doi.org/10.3390/nano9121736
- Asai, S., Miyasaka, F., Nomura, K., Ogino, Y., Tanaka, M., Shigeta, M., Yamane, S. (2020). Recent Progresses of Welding and Joining Engineering. Journal of the Japan Welding Society, 89 (5), 322–335. doi: https://doi.org/10.2207/jjws.89.322
- Shigeta, M. (2018). Modeling and simulation of a turbulent‐like thermal plasma jet for nanopowder production. IEEJ Transactions on Electrical and Electronic Engineering, 14 (1), 16–28. doi: https://doi.org/10.1002/tee.22761
- Shigeta, M. (2020). Simulating Turbulent Thermal Plasma Flows for Nanopowder Fabrication. Plasma Chemistry and Plasma Processing, 40 (3), 775–794. doi: https://doi.org/10.1007/s11090-020-10060-8
- Porrang, S., Rahemi, N., Davaran, S., Mahdavi, M., Hassanzadeh, B., Gholipour, A. M. (2021). Direct surface modification of mesoporous silica nanoparticles by DBD plasma as a green approach to prepare dual-responsive drug delivery system. Journal of the Taiwan Institute of Chemical Engineers, 123, 47–58. doi: https://doi.org/10.1016/j.jtice.2021.05.024
- Banerjee, S., Adhikari, E., Sapkota, P., Sebastian, A., Ptasinska, S. (2020). Atmospheric Pressure Plasma Deposition of TiO2: A Review. Materials, 13 (13), 2931. doi: https://doi.org/10.3390/ma13132931
- Dasgupta, D., Peddi, S., Saini, D. K., Ghosh, A. (2022). Mobile Nanobots for Prevention of Root Canal Treatment Failure. Advanced Healthcare Materials, 11 (14). doi: https://doi.org/10.1002/adhm.202200232
- Kumaresan, L., Shanmugavelayutham, G., Surendran, S., Sim, U. (2022). Thermal plasma arc discharge method for high-yield production of hexagonal AlN nanoparticles: synthesis and characterization. Journal of the Korean Ceramic Society, 59 (3), 338–349. doi: https://doi.org/10.1007/s43207-021-00177-7
- Huang, Y., Li, Q., Xue, X., Xu, H., Huang, J., Fan, D. (2022). Electrostatic probe analysis of SiO2 activating flux powders transition behavior in Powder Pool Coupled Activating TIG alternating current arc plasma for aluminum alloy. Journal of Manufacturing Processes, 84, 600–609. doi: https://doi.org/10.1016/j.jmapro.2022.10.029
- Conversano, R. W., Lobbia, R. B., Kerber, T. V., Tilley, K. C., Goebel, D. M., Reilly, S. W. (2019). Performance characterization of a low-power magnetically shielded Hall thruster with an internally-mounted hollow cathode. Plasma Sources Science and Technology, 28 (10), 105011. doi: https://doi.org/10.1088/1361-6595/ab47de
- Fauchais, P. (1984). Applications physico-chimiques des plasmas d’arc. Revue de Physique Appliquée, 19 (12), 1013–1045. doi: https://doi.org/10.1051/rphysap:0198400190120101300
- Hassouba, M. A., Galaly, A. R., Rashed, U. M. (2013). Analysis of cylindrical Langmuir probe using experiment and different theories. Plasma Physics Reports, 39 (3), 255–262. doi: https://doi.org/10.1134/s1063780x13030033
- Honglertkongsakul, K., Chaiyakun, S., Witit-anun, N., Kongsri, W., Limsuwan, P. (2012). Single Langmuir Probe Measurements in an Unbalanced Magnetron Sputtering System. Procedia Engineering, 32, 962–968. doi: https://doi.org/10.1016/j.proeng.2012.02.039
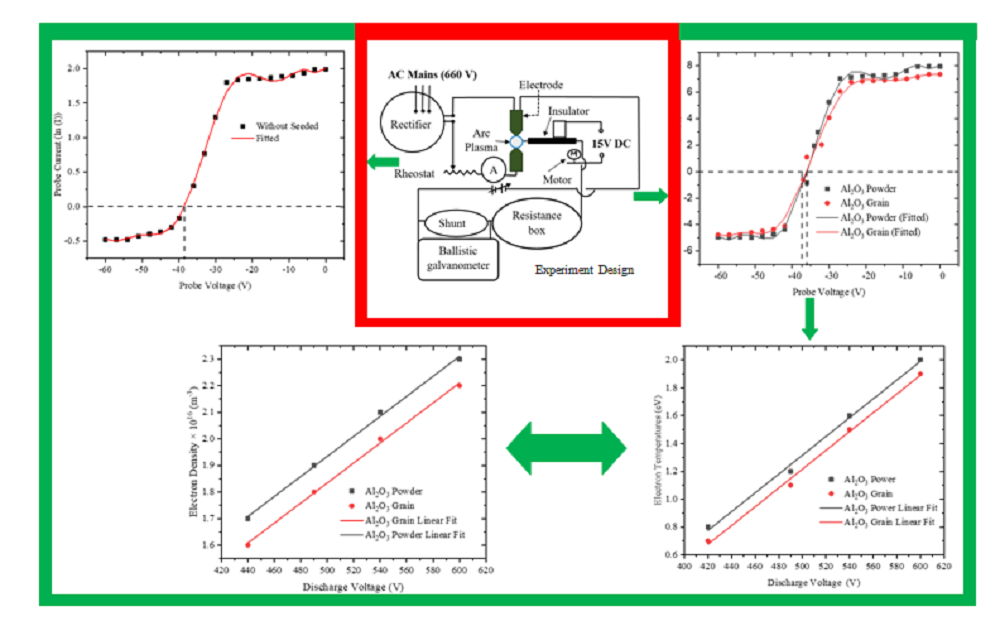
Downloads
Published
How to Cite
Issue
Section
License
Copyright (c) 2023 Vijay Kumar Jha, Lekha Nath Mishra, Bijoyendra Narayan, Saddam Husain Dhobi, Arun Kumar Shah, Susmita Jha

This work is licensed under a Creative Commons Attribution 4.0 International License.
The consolidation and conditions for the transfer of copyright (identification of authorship) is carried out in the License Agreement. In particular, the authors reserve the right to the authorship of their manuscript and transfer the first publication of this work to the journal under the terms of the Creative Commons CC BY license. At the same time, they have the right to conclude on their own additional agreements concerning the non-exclusive distribution of the work in the form in which it was published by this journal, but provided that the link to the first publication of the article in this journal is preserved.
A license agreement is a document in which the author warrants that he/she owns all copyright for the work (manuscript, article, etc.).
The authors, signing the License Agreement with TECHNOLOGY CENTER PC, have all rights to the further use of their work, provided that they link to our edition in which the work was published.
According to the terms of the License Agreement, the Publisher TECHNOLOGY CENTER PC does not take away your copyrights and receives permission from the authors to use and dissemination of the publication through the world's scientific resources (own electronic resources, scientometric databases, repositories, libraries, etc.).
In the absence of a signed License Agreement or in the absence of this agreement of identifiers allowing to identify the identity of the author, the editors have no right to work with the manuscript.
It is important to remember that there is another type of agreement between authors and publishers – when copyright is transferred from the authors to the publisher. In this case, the authors lose ownership of their work and may not use it in any way.