Patterns in the electrochemical synthesis of thin-film photocatalytic materials based on titanium heterooxide compounds
DOI:
https://doi.org/10.15587/1729-4061.2022.269942Keywords:
plasma-electrolyte oxidation, titanium heterooxides, heterooxidic photocatalyst, photocatalyst morphology, azo dye, dopantAbstract
This paper reports a study the into processes of plasma-electrolyte formation of heterooxidic coatings on titanium alloys for the photocatalytic destruction of natural and man-made toxicants. The synthesis of coatings was carried out from aqueous solutions of diphosphates under a galvanostatic mode. For a quantitative description of photocatalytic reactions, the constants of the reaction rate kf from the linearized dependences ln(Ct/C0), where Ct is the current, C0 is the initial concentration of the reagent, were calculated. The morphology of the coating surface was investigated by atomic force microscopy, and the results were visualized by reconstruction of the relief in the form of 2D and 3D topographic maps. Morphological features of coatings made of titanium oxide (IV) and heterooxidic composites containing oxides of transition metals were analyzed. It is shown that the specific surface area remains an effective factor in regulating the photocatalytic activity of coatings. The establishment of the morphology of heterooxidic composites, as well as methods for controlling this parameter of the target product, is a constant component of the systematic study of such materials in determining their functional properties. It has been established that compared to oxide-titanium coatings whose surface layers are characterized by a toroidal mesostructure, heterooxidic compositions have a more developed surface, which has a positive effect on their functional properties. Subsequent heat treatment similarly affects the properties of the coating. The speed constants of the photocatalytic decomposition of the methyl yellow-hot azo dye were used to rank coatings of different composition according to their functional properties. Coatings from TiO2·ZnO showed the highest catalytic activity among the materials studied – kf is 5.26 10-3 min-1, which is several times higher than the corresponding value for TiO2
References
- Karakurkchi, A., Sakhnenko, M., Ved, M., Galak, A., Petrukhin, S. (2017). Application of oxide-metallic catalysts on valve metals for ecological catalysis. Eastern-European Journal of Enterprise Technologies, 5 (10 (89)), 12–18. doi: https://doi.org/10.15587/1729-4061.2017.109885
- Khimach, N. Yu., Polunkin, Ye. V. (2012). Nanostrukturovani katalizatory. Katalyz y neftekhymyia, 21, 86–98.
- Ved, M. V. (2017). Functional mixed cobalt and aluminum oxide coatings for environmental safety. Functional Materials, 24 (2), 005–310. doi: https://doi.org/10.15407/fm24.02.303
- Ved, M. V., Sakhnenko, M. D. (2010). Katalitychni ta zakhysni pokryttia splavamy i skladnymy oksydamy: elektrokhimichnyi syntez, prohnozuvannia vlastyvostei. Kharkiv: NTU „KhPI”, 272.
- Halak, O., Menshov, S. (2019). The use of photocatalytic technology for the disintegration of hazardous chemical substances. International Scientific Conference. doi: https://doi.org/10.30525/978-9934-588-11-2_9
- Kadhim S. H. (2016). Preparation and Characterization of Pure and Na2O Doped Co3O4 Spinel Supported Catalyst for Photocatalytic Degradation of Reactive Yellow Dye 145. International Journal of ChemTech Research, 9 (12), 754–766. Available at: https://www.sphinxsai.com/2016/ch_vol9_no12/2/(754-766)V9N12CT.pdf
- Qu, Y., Duan, X. (2013). Progress, challenge and perspective of heterogeneous photocatalysts. Chem. Soc. Rev., 42 (7), 2568–2580. doi: https://doi.org/10.1039/c2cs35355e
- Khairy, M., Zakaria, W. (2014). Effect of metal-doping of TiO2 nanoparticles on their photocatalytic activities toward removal of organic dyes. Egyptian Journal of Petroleum, 23 (4), 419–426. doi: https://doi.org/10.1016/j.ejpe.2014.09.010
- Iurascu, B., Siminiceanu, I., Vione, D., Vicente, M. A., Gil, A. (2009). Phenol degradation in water through a heterogeneous photo-Fenton process catalyzed by Fe-treated laponite. Water Research, 43 (5), 1313–1322. doi: https://doi.org/10.1016/j.watres.2008.12.032
- Grishina, E. P., Kudryakova, N. O., Rumyantsev, P. A., Zhirov, A. V., Gladiy, Yu. P., Belkin, P. N. (2013). Photoelectrochemical properties of oxide films formed by anode plasma electrolytic oxidation on titanium in water solutions. Surface Engineering and Applied Electrochemistry, 49 (1), 83–90. doi: https://doi.org/10.3103/s1068375512060026
- Marcì, G., Augugliaro, V., López-Muñoz, M. J., Martín, C., Palmisano, L., Rives, V. et al. (2001). Preparation Characterization and Photocatalytic Activity of Polycrystalline ZnO/TiO2 Systems. 2. Surface, Bulk Characterization, and 4-Nitrophenol Photodegradation in Liquid−Solid Regime. The Journal of Physical Chemistry B, 105 (5), 1033–1040. doi: https://doi.org/10.1021/jp003173j
- Meng, T., Xie, P., Qin, H., Liu, H., Hua, W., Li, X., Ma, Z. (2016). Fe2O3/SiO2 nanowires formed by hydrothermally transforming SiO2 spheres in the presence of Fe3+: Synthesis, characterization, and catalytic properties. Journal of Molecular Catalysis A: Chemical, 421, 109–116. doi: https://doi.org/10.1016/j.molcata.2016.05.017
- Kandy, M. M., Gaikar, V. G. (2018). Photocatalytic reduction of CO2 using CdS nanorods on porous anodic alumina support. Materials Research Bulletin, 102, 440–449. doi: https://doi.org/10.1016/j.materresbull.2018.02.054
- He, J., Luo, Q., Cai, Q. Z., Li, X. W., Zhang, D. Q. (2011). Microstructure and photocatalytic properties of WO3/TiO2 composite films by plasma electrolytic oxidation. Materials Chemistry and Physics, 129 (1-2), 242–248. doi: https://doi.org/10.1016/j.matchemphys.2011.04.011
- Paramasivam, I., Jha, H., Liu, N., Schmuki, P. (2012). A Review of Photocatalysis using Self-organized TiO2Nanotubes and Other Ordered Oxide Nanostructures. Small, 8 (20), 3073–3103. doi: https://doi.org/10.1002/smll.201200564
- Vasilyeva, M. S., Rudnev, V. S., Tarabrina, D. A. (2017). Photocatalytic properties of Zn- and Cd-containing oxide layers on titanium formed by plasma electrolytic oxidation. Protection of Metals and Physical Chemistry of Surfaces, 53 (4), 711–715. doi: https://doi.org/10.1134/s2070205117040232
- Zaleska, A. (2008). Doped-TiO2: A Review. Recent Patents on Engineering, 2 (3), 157–164. doi: https://doi.org/10.2174/187221208786306289
- Karakurkchi, A. V. (2015). Functional properties of multicomponent galvanic alloys of iron with molybdenum and tungsten. Functional Materials, 22 (2), 181–187. doi: https://doi.org/10.15407/fm22.02.181
- Zhang, F., Wang, X., Liu, H., Liu, C., Wan, Y., Long, Y., Cai, Z. (2019). Recent Advances and Applications of Semiconductor Photocatalytic Technology. Applied Sciences, 9 (12), 2489. doi: https://doi.org/10.3390/app9122489
- Xiao, F.-X. (2012). Construction of Highly Ordered ZnO–TiO2 Nanotube Arrays (ZnO/TNTs) Heterostructure for Photocatalytic Application. ACS Applied Materials & Interfaces, 4 (12), 7055–7063. doi: https://doi.org/10.1021/am302462d
- Fujishima, A., Zhang, X. (2006). Titanium dioxide photocatalysis: present situation and future approaches. Comptes Rendus Chimie, 9 (5-6), 750–760. doi: https://doi.org/10.1016/j.crci.2005.02.055
- Asahi, R., Morikawa, T., Ohwaki, T., Aoki, K., Taga, Y. (2001). Visible-Light Photocatalysis in Nitrogen-Doped Titanium Oxides. Science, 293 (5528), 269–271. doi: https://doi.org/10.1126/science.1061051
- Irie, H., Watanabe, Y., Hashimoto, K. (2003). Nitrogen-Concentration Dependence on Photocatalytic Activity of TiO2-xNx Powders. The Journal of Physical Chemistry B, 107 (23), 5483–5486. doi: https://doi.org/10.1021/jp030133h
- Ihara, T. (2003). Visible-light-active titanium oxide photocatalyst realized by an oxygen-deficient structure and by nitrogen doping. Applied Catalysis B: Environmental, 42 (4), 403–409. doi: https://doi.org/10.1016/s0926-3373(02)00269-2
- Kudo, A. (2003). Photocatalyst materials for water splitting. Catalysis Surveys from Asia, 7, 31–38. doi: https://doi.org/10.1023/A:1023480507710
- Prieto, J. P., Béjar, M. G. (Eds.) (2019). Photoactive inorganic nanoparticles. Surface composition and nanosystem functionality. Elsevier. doi: https://doi.org/10.1016/c2017-0-01254-5
- Ved’, M. V., Sakhnenko, M. D., Bohoyavlens’ka, O. V., Nenastina, T. O. (2008). Modeling of the surface treatment of passive metals. Materials Science, 44 (1), 79–86. doi: https://doi.org/10.1007/s11003-008-9046-6
- Sakhnenko, N. D., Ved, M. V., Karakurkchi, A. V. (2017). Nanoscale Oxide PEO Coatings Forming from Diphosphate Electrolytes. Nanophysics, Nanomaterials, Interface Studies, and Applications, 507–531. doi: https://doi.org/10.1007/978-3-319-56422-7_38
- Yan, G., Zhang, M., Hou, J., Yang, J. (2011). Photoelectrochemical and photocatalytic properties of N+S co-doped TiO2 nanotube array films under visible light irradiation. Materials Chemistry and Physics, 129 (1-2), 553–557. doi: https://doi.org/10.1016/j.matchemphys.2011.04.063
- Yar-Mukhamedova, G. Sh., Sakhnenko, N. D., Ved, M. V. (2020). Nanocomposite electrolytic coatings with defined functional properties. Almaty: Kazakh University, 180.
- Lim, S. Y., Law, C. S., Liu, L., Markovic, M., Hedrich, C., Blick, R. H. et al. (2019). Electrochemical Engineering of Nanoporous Materials for Photocatalysis: Fundamentals, Advances, and Perspectives. Catalysts, 9 (12), 988. doi: https://doi.org/10.3390/catal9120988
- Luo, Q., Cai, Q., Li, X., Chen, X. (2014). Characterization and photocatalytic activity of large-area single crystalline anatase TiO2 nanotube films hydrothermal synthesized on Plasma electrolytic oxidation seed layers. Journal of Alloys and Compounds, 597, 101–109. doi: https://doi.org/10.1016/j.jallcom.2014.01.216
- Herrmann, J.-M. (2005). Heterogeneous photocatalysis: state of the art and present applications In honor of Pr. R.L. Burwell Jr. (1912–2003), Former Head of Ipatieff Laboratories, Northwestern University, Evanston (Ill). Topics in Catalysis, 34 (1-4), 49–65. doi: https://doi.org/10.1007/s11244-005-3788-2
- Vinu, R., Madras, G. (2010). Environmental remediation by photocatalysis. Journal of the Indian Institute of Science, 90 (2), 189–230. Available at: http://journal.iisc.ernet.in/index.php/iisc/article/view/95/92
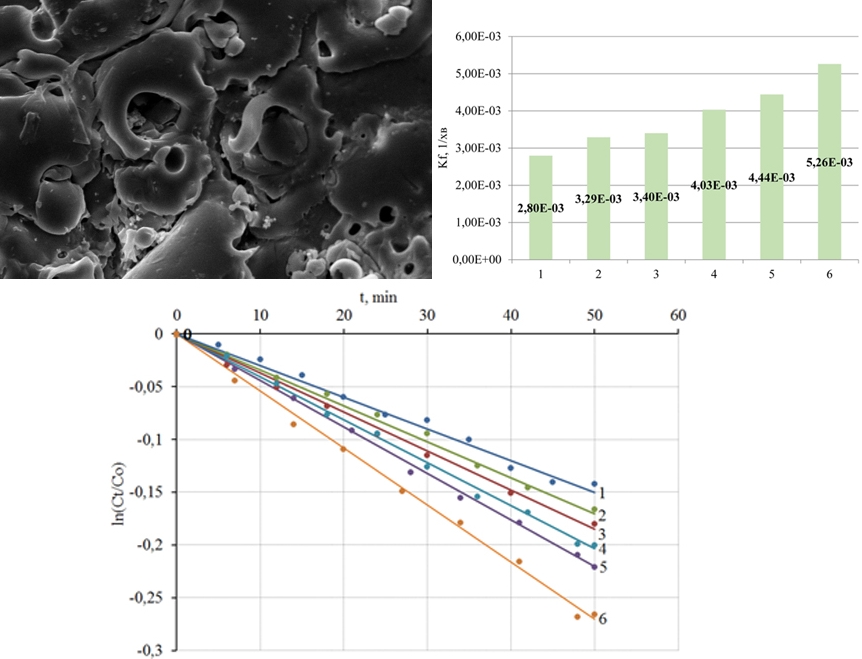
Downloads
Published
How to Cite
Issue
Section
License
Copyright (c) 2022 Mykola Sakhnenko, Iryna Stepanova, Alla Korogodskaya, Ann Karakurkchi, Olena Skrypnyk, Anatoly Dzheniuk, Oleksandr Halak

This work is licensed under a Creative Commons Attribution 4.0 International License.
The consolidation and conditions for the transfer of copyright (identification of authorship) is carried out in the License Agreement. In particular, the authors reserve the right to the authorship of their manuscript and transfer the first publication of this work to the journal under the terms of the Creative Commons CC BY license. At the same time, they have the right to conclude on their own additional agreements concerning the non-exclusive distribution of the work in the form in which it was published by this journal, but provided that the link to the first publication of the article in this journal is preserved.
A license agreement is a document in which the author warrants that he/she owns all copyright for the work (manuscript, article, etc.).
The authors, signing the License Agreement with TECHNOLOGY CENTER PC, have all rights to the further use of their work, provided that they link to our edition in which the work was published.
According to the terms of the License Agreement, the Publisher TECHNOLOGY CENTER PC does not take away your copyrights and receives permission from the authors to use and dissemination of the publication through the world's scientific resources (own electronic resources, scientometric databases, repositories, libraries, etc.).
In the absence of a signed License Agreement or in the absence of this agreement of identifiers allowing to identify the identity of the author, the editors have no right to work with the manuscript.
It is important to remember that there is another type of agreement between authors and publishers – when copyright is transferred from the authors to the publisher. In this case, the authors lose ownership of their work and may not use it in any way.