Implementation of radar cross-sections model for targets with different scattering centers
DOI:
https://doi.org/10.15587/1729-4061.2022.265089Keywords:
radar cross-section (RCS), target scattering, point targets, measured targets’ profiles, extended targetsAbstract
Target scattering or reflection is used by radar systems to identify and detect targets. The larger the echo that was returned to the radar receiver, the superior the signal-to-noise ratio and the greater the likelihood of detection. The radar cross-section (RCS) determines the quantity of energy reflected from a target in radar systems. This work shows new modeling for radar targets with growing stages of fidelity. We introduce the RCS concept for straightforward point targets and extend this into additional complex states of targets with several scattering midpoints. In addition, we discuss the modeling of fluctuations in RCS with time and briefly consider the case of the polarized signal. Because the receiver and transmitter are co-located, the effort focuses on narrowband mono-static radar techniques. The RCS value changes between scans. We simulate the replicated power of a sent signal over 10,000 scanning over unit incident signals, assuming that the signal illuminates the target just once each dwell. The target is modeled by four scatterers that are placed at four square vertices. All scatterers are cylindrical-point targets on a 0.5-meter square XY plane without losing generality. The acquired results demonstrated how to generate target echoes while accounting for statistical fluctuations. From the relation between RCS and elevation angle variations for cylindrical targets, the obtained result demonstrated that the first two outputs are the same and confirmed that there is no reliance on azimuth angle. The comparison between wideband and narrowband RCS patterns demonstrated that the RCS profiles of the target-matched shallower nulls for azimuth direction are in the range of (40–50) degrees at zero elevation for 4 scatterers’ extended targets.
Supporting Agency
- The authors would like to express their deepest gratitude to the University of Technology Baghdad-Iraq for their support to complete this research.
References
- Semkin, V., Haarla, J., Pairon, T., Slezak, C., Rangan, S., Viikari, V., Oestges, C. (2020). Analyzing Radar Cross Section Signatures of Diverse Drone Models at mmWave Frequencies. IEEE Access, 8, 48958–48969. doi: https://doi.org/10.1109/access.2020.2979339
- Tian, Z.-F., Wu, D., Hu, T. (2022). Theoretical study of single-photon quantum radar cross-section of cylindrical curved surface. Acta Physica Sinica, 71 (3), 034204. doi: https://doi.org/10.7498/aps.71.20211295
- Sengupta, S., Council, H., Jackson, D. R., Onofrei, D. (2020). Active Radar Cross Section Reduction of an Object Using Microstrip Antennas. Radio Science, 55 (2). doi: https://doi.org/10.1029/2019rs006939
- Zhang, T., Zeng, H., Chen, R. (2019). Simulation of Quantum Radar Cross Section for Electrically Large Targets With GPU. IEEE Access, 7, 154260–154267. doi: https://doi.org/10.1109/access.2019.2947738
- Rajyalakshmi, P., Raju, G. S. N. (2011). Characteristics of Radar Cross Section with Different Objects. International Journal of Electronics and Communication Engineeringб 4 (2), 205–216. Available at: https://www.ripublication.com/irph/ijece/ijecev4n2__6.pdf
- Shi, W., Zhang, X., Shen, Y., Chen, H., Yu, Z. (2021). RCS Estimation and Synthesis of Typical Traffic Participants. 2021 Photonics & Electromagnetics Research Symposium (PIERS). doi: https://doi.org/10.1109/piers53385.2021.9694942
- Dallmann, T., Heberling, D. (2014). Discrimination of scattering mechanisms via polarimetric rcs imaging [measurements corner]. IEEE Antennas and Propagation Magazine, 56 (3), 154–165. doi: https://doi.org/10.1109/map.2014.6867696
- Alberga, V. (2004). Volume decorrelation effects in polarimetric SAR interferometry. IEEE Transactions on Geoscience and Remote Sensing, 42 (11), 2467–2478. doi: https://doi.org/10.1109/tgrs.2004.837330
- Shijer, S. S., Sabry, A. H. (2021). Analysis of performance parameters for wireless network using switching multiple access control method. Eastern-European Journal of Enterprise Technologies, 4 (9 (112)), 6–14. doi: https://doi.org/10.15587/1729-4061.2021.238457
- Al-Shoukry, S., M. Jawad, B. J., Musa, Z., Sabry, A. H. (2022). Development of predictive modeling and deep learning classification of taxi trip tolls. Eastern-European Journal of Enterprise Technologies, 3 (3 (117)), 6–12. doi: https://doi.org/10.15587/1729-4061.2022.259242
- Li, M., Xiang, Y., Chen, X., Wang, Y., Lu, Y., Ding, Z. (2019). Altitude ambiguity suppression method based on dual‐frequency interfering and multi‐frequency averaging for sparse baseline TomoSAR. Electronics Letters, 55 (22), 1194–1196. doi: https://doi.org/10.1049/el.2019.2198
- Ning, C., Lü, M., Gao, C., Wan, H. (2021). Clutter Reduction Method for Radar Cross Section (RCS) Measurement Based on InSAR Principle. Beijing Ligong Daxue Xuebao/Transaction of Beijing Institute of Technology, 41 (08). doi: https://doi.org/10.15918/j.tbit1001-0645.2021.024
- Margarit, G., Mallorqui, J. J., Fortuny-Guasch, J., Lopez-Martinez, C. (2009). Exploitation of Ship Scattering in Polarimetric SAR for an Improved Classification Under High Clutter Conditions. IEEE Transactions on Geoscience and Remote Sensing, 47 (4), 1224–1235. doi: https://doi.org/10.1109/tgrs.2008.2008721
- Lee, K.-C. (2019). Radar target recognition by frequency-diversity rcs together with kernel scatter difference discrimination. Progress In Electromagnetics Research M, 87, 137–145. doi: https://doi.org/10.2528/pierm19101201
- Deep, Y., Held, P., Ram, S. S., Steinhauser, D., Gupta, A., Gruson, F. et. al. (2020). Radar cross‐sections of pedestrians at automotive radar frequencies using ray tracing and point scatterer modelling. IET Radar, Sonar & Navigation, 14(6), 833–844. doi: https://doi.org/10.1049/iet-rsn.2019.0471
- Baldauf, J., Lee, S.-W., Lin, L., Jeng, S.-K., Scarborough, S. M., Yu, C. L. (1991). High frequency scattering from trihedral corner reflectors and other benchmark targets: SBR versus experiment. IEEE Transactions on Antennas and Propagation, 39 (9), 1345–1351. doi: https://doi.org/10.1109/8.99043
- Skolnik, M. I. (2008). Radar Handbook. McGraw Hill, 1328.
- Youssef, N. N. (1989). Radar cross section of complex targets. Proceedings of the IEEE, 77 (5), 722–734. doi: https://doi.org/10.1109/5.32062
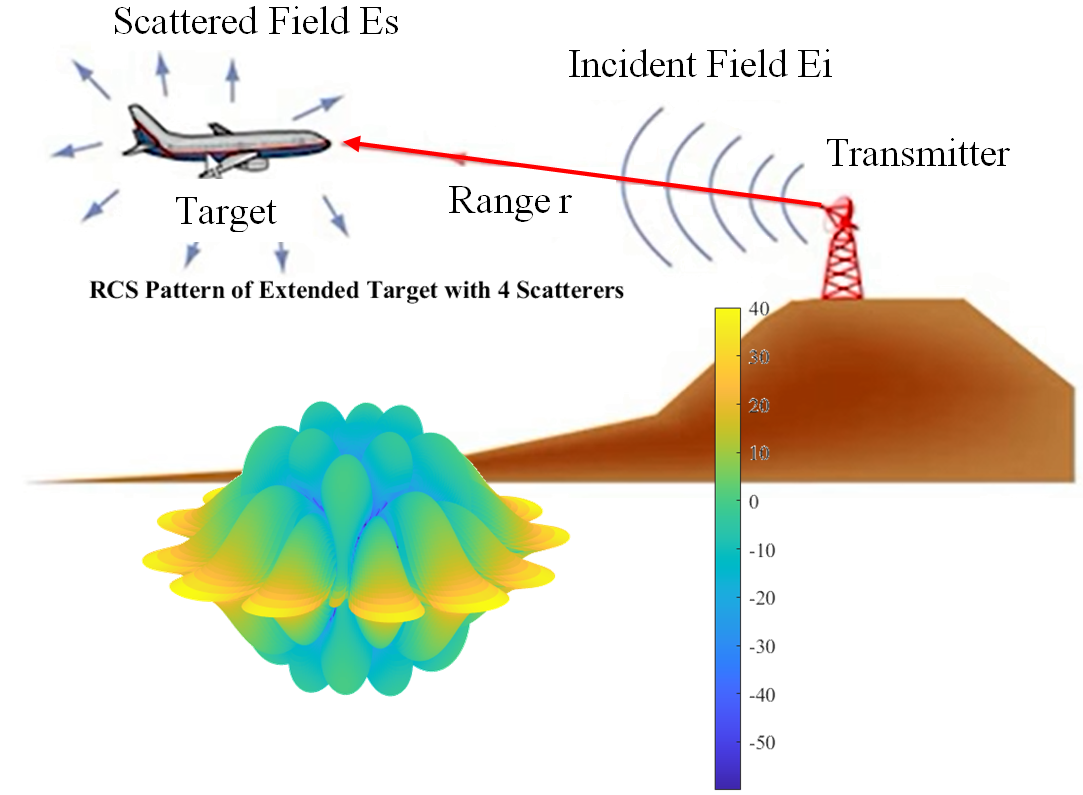
Downloads
Published
How to Cite
Issue
Section
License
Copyright (c) 2022 Sameir Aziez, Ekhlas Hamza, Fadia Hummadi, Ahmad Sabry

This work is licensed under a Creative Commons Attribution 4.0 International License.
The consolidation and conditions for the transfer of copyright (identification of authorship) is carried out in the License Agreement. In particular, the authors reserve the right to the authorship of their manuscript and transfer the first publication of this work to the journal under the terms of the Creative Commons CC BY license. At the same time, they have the right to conclude on their own additional agreements concerning the non-exclusive distribution of the work in the form in which it was published by this journal, but provided that the link to the first publication of the article in this journal is preserved.
A license agreement is a document in which the author warrants that he/she owns all copyright for the work (manuscript, article, etc.).
The authors, signing the License Agreement with TECHNOLOGY CENTER PC, have all rights to the further use of their work, provided that they link to our edition in which the work was published.
According to the terms of the License Agreement, the Publisher TECHNOLOGY CENTER PC does not take away your copyrights and receives permission from the authors to use and dissemination of the publication through the world's scientific resources (own electronic resources, scientometric databases, repositories, libraries, etc.).
In the absence of a signed License Agreement or in the absence of this agreement of identifiers allowing to identify the identity of the author, the editors have no right to work with the manuscript.
It is important to remember that there is another type of agreement between authors and publishers – when copyright is transferred from the authors to the publisher. In this case, the authors lose ownership of their work and may not use it in any way.