The mechanism of pH recalibration by dissolved oxygen in alkaline modified aquaculture seawater
DOI:
https://doi.org/10.15587/1729-4061.2022.266012Keywords:
aeration treatment, molecular mechanism, dissolved oxygen, pH recalibration, aquaculture seawaterAbstract
The balance of dissolved oxygen and pH levels is paramount in aquaculture, as a media for cultivating aquatic organisms under controlled conditions. An imbalance in both oxygen and pH could severely harm the cultured aquatic organisms. Various strategies are used to prevent hypoxia and maintain the pH level of the culture. Interestingly, hypoxia or deprivation of oxygen supply in aquaculture was often reported to co-occur with the seawater acidification. Despite that, there was no evidence that the O2 level was directly linked to pH changes. Thus, the existing treatment strategies are separated between O2 and pH maintenances, which often inflate cost and cause environmental burden due to the use of synthetic chemicals. This study was conducted to observe the mechanism and effect of the O2 addition to aquaculture seawater in molecular level when the pH value of the water was modified. The understanding of the mechanism may lead to an alternative to the harmful aquaculture treatments. The molecular mechanics analysis was applied to examine the mechanism of pH adjustment in non-aerated and aerated seawater. The results indicated that O2 accelerated the pH recalibration of seawater, particularly in the alkaline modified samples compared to the acid modified samples. Mechanical simulations further showed the repulsion between and O2 causes vibration which shortens OH bond by 17.71 % while elongates O-O bond by 1.00 %. Additionally, the spin coupling between OH- and O2 promotes global energy transfer which stimulates the vibration of the alkaline modified water system. Together, those mechanisms enabled the pH value to return to the baseline. These findings contribute a molecular mechanism view of aquaculture pH maintenance in the presence of O2, as well as revisiting the use of aeration in aquaculture treatment
Supporting Agency
- The authors are deeply grateful to Yogita A. D. Susanti, Zulkisam Pramudia, Abdul Azis Amin, and Adi Tiya Yanuar from Microbial Resources and Biotechnology research group of the Postgraduate School of Brawijaya University for their assistance.
References
- Henriksson, P. J. G., Tran, N., Mohan, C. V., Chan, C. Y., Rodriguez, U.-P., Suri, S. et. al. (2017). Indonesian aquaculture futures – Evaluating environmental and socioeconomic potentials and limitations. Journal of Cleaner Production, 162, 1482–1490. doi: https://doi.org/10.1016/j.jclepro.2017.06.133
- Rimmer, M. A., Larson, S., Lapong, I., Purnomo, A. H., Pong-Masak, P. R., Swanepoel, L., Paul, N. A. (2021). Seaweed Aquaculture in Indonesia Contributes to Social and Economic Aspects of Livelihoods and Community Wellbeing. Sustainability, 13 (19), 10946. doi: https://doi.org/10.3390/su131910946
- Zhang, P., Zhang, X., Li, J., Huang, G. (2006). The effects of body weight, temperature, salinity, pH, light intensity and feeding condition on lethal DO levels of whiteleg shrimp, Litopenaeus vannamei (Boone, 1931). Aquaculture, 256 (1-4), 579–587. doi: https://doi.org/10.1016/j.aquaculture.2006.02.020
- Thulasi, D., Muralidhar, M., Saraswathy, R. (2020). Effect of sulphide in Pacific white shrimp Penaeus vannamei under varying oxygen and pH levels. Aquaculture Research, 51 (6), 2389–2399. doi: https://doi.org/10.1111/are.14582
- Chan, F., Barth, J., Kroeker, K., Lubchenco, J., Menge, B. (2019). The Dynamics and Impact of Ocean Acidification and Hypoxia: Insights from Sustained Investigations in the Northern California Current Large Marine Ecosystem. Oceanography, 32 (3), 62–71. doi: https://doi.org/10.5670/oceanog.2019.312
- Zeebe, R. E. (2012). History of Seawater Carbonate Chemistry, Atmospheric CO2, and Ocean Acidification. Annual Review of Earth and Planetary Sciences, 40 (1), 141–165. doi: https://doi.org/10.1146/annurev-earth-042711-105521
- Anggayasti, W. L., Mancera, R. L., Bottomley, S., Helmerhorst, E. (2017). The self-association of HMGB1 and its possible role in the binding to DNA and cell membrane receptors. FEBS Letters, 591 (2), 282–294. doi: https://doi.org/10.1002/1873-3468.12545
- Ulaje, S. A., Lluch-Cota, S. E., Sicard, M. T., Ascencio, F., Cruz-Hernández, P., Racotta, I. S., Rojo-Arreola, L. (2020). Litopenaeus vannamei oxygen consumption and HSP gene expression at cyclic conditions of hyperthermia and hypoxia. Journal of Thermal Biology, 92, 102666. doi: https://doi.org/10.1016/j.jtherbio.2020.102666
- Sultana, T., Haque, M., Salam, M., Alam, M. (2017). Effect of aeration on growth and production of fish in intensive aquaculture system in earthen ponds. Journal of the Bangladesh Agricultural University, 15 (1), 113–122. doi: https://doi.org/10.3329/jbau.v15i1.33536
- Tanveer, M., Roy, S. M., Vikneswaran, M., Renganathan, P., Balasubramanian, P. (2018). Surface aeration systems for application in aquaculture: A review. International Journal of Fisheries and Aquatic Studies, 6 (5), 342–347. Available at: https://www.fisheriesjournal.com/archives/2018/vol6issue5/PartE/6-5-23-591.pdf
- Wang, X., Shang, Y., Kong, H., Hu, M., Yang, J., Deng, Y., Wang, Y. (2020). Combined effects of ocean acidification and hypoxia on the early development of the thick shell mussel Mytilus coruscus. Helgoland Marine Research, 74 (1). doi: https://doi.org/10.1186/s10152-020-0535-9
- Sylvain, F.-É., Cheaib, B., Llewellyn, M., Gabriel Correia, T., Barros Fagundes, D. et. al. (2016). pH drop impacts differentially skin and gut microbiota of the Amazonian fish tambaqui (Colossoma macropomum). Scientific Reports, 6 (1). doi: https://doi.org/10.1038/srep32032
- George, M. N., Andino, J., Huie, J., Carrington, E. (2019). Microscale pH and Dissolved Oxygen Fluctuations within Mussel Aggregations and Their Implications for Mussel Attachment and Raft Aquaculture. Journal of Shellfish Research, 38 (3), 795. doi: https://doi.org/10.2983/035.038.0329
- Carstensen, J., Duarte, C. M. (2019). Drivers of pH Variability in Coastal Ecosystems. Environmental Science & Technology, 53 (8), 4020–4029. doi: https://doi.org/10.1021/acs.est.8b03655
- Hlordzi, V., Kuebutornye, F. K. A., Afriyie, G., Abarike, E. D., Lu, Y., Chi, S., Anokyewaa, M. A. (2020). The use of Bacillus species in maintenance of water quality in aquaculture: A review. Aquaculture Reports, 18, 100503. doi: https://doi.org/10.1016/j.aqrep.2020.100503
- Irawan, Y., Fonda, H., Sabna, E., Febriani, A. (2021). Intelligent Quality Control of Shrimp Aquaculture Based On Real-Time System and IoT Using Mobile Device. International Journal of Engineering Trends and Technology, 69 (4), 49–56. doi: https://doi.org/10.14445/22315381/ijett-v69i4p208
- Diez, A. (2021). SiSyPHE: A Python package for the Simulation of Systems of interacting mean-field Particles with High Efficiency. Journal of Open Source Software, 6 (65), 3653. doi: https://doi.org/10.21105/joss.03653
- D’Orsogna, M. R., Chuang, Y. L., Bertozzi, A. L., Chayes, L. S. (2006). Self-Propelled Particles with Soft-Core Interactions: Patterns, Stability, and Collapse. Physical Review Letters, 96 (10). doi: https://doi.org/10.1103/physrevlett.96.104302
- Ahmed, A. A. M. (2017). Prediction of dissolved oxygen in Surma River by biochemical oxygen demand and chemical oxygen demand using the artificial neural networks (ANNs). Journal of King Saud University - Engineering Sciences, 29 (2), 151–158. doi: https://doi.org/10.1016/j.jksues.2014.05.001
- Minaev, B. F. (2017). Spin-orbit coupling mechanism of singlet oxygen a1Δg quenching by solvent vibrations. Chemical Physics, 483-484, 84–95. doi: https://doi.org/10.1016/j.chemphys.2016.11.012
- Hosoya, A., Maruyama, K., Shikano, Y. (2015). Operational derivation of Boltzmann distribution with Maxwell’s demon model. Scientific Reports, 5 (1). doi: https://doi.org/10.1038/srep17011
- Hua, Z., Tian, C., Qiu, Z., Li, Y., Tian, X., Wang, M., Li, E. (2018). An investigation on NO2 sensing mechanism and shielding behavior of WO3 nanosheets. Sensors and Actuators B: Chemical, 259, 250–257. doi: https://doi.org/10.1016/j.snb.2017.12.016
- Golse, F. (2016). On the Dynamics of Large Particle Systems in the Mean Field Limit. Lecture Notes in Applied Mathematics and Mechanics, 1–144. doi: https://doi.org/10.1007/978-3-319-26883-5_1
- Miles, C. E., Zhu, J., Mogilner, A. (2022). Mechanical Torque Promotes Bipolarity of the Mitotic Spindle Through Multi-centrosomal Clustering. Bulletin of Mathematical Biology, 84 (2). doi: https://doi.org/10.1007/s11538-021-00985-2
- Grozdanov, S., Schalm, K., Scopelliti, V. (2019). Kinetic theory for classical and quantum many-body chaos. Physical Review E, 99 (1). doi: https://doi.org/10.1103/physreve.99.012206
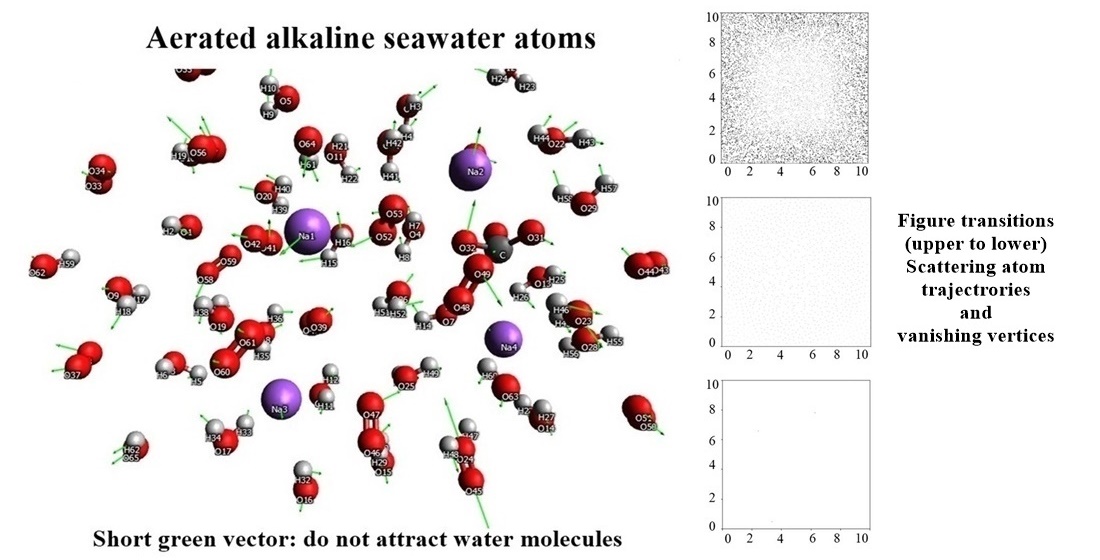
Downloads
Published
How to Cite
Issue
Section
License
Copyright (c) 2022 Wresti L. Anggayasti, Willy Satrio N., I Nyoman Gede Wardana, Andi Kurniawan

This work is licensed under a Creative Commons Attribution 4.0 International License.
The consolidation and conditions for the transfer of copyright (identification of authorship) is carried out in the License Agreement. In particular, the authors reserve the right to the authorship of their manuscript and transfer the first publication of this work to the journal under the terms of the Creative Commons CC BY license. At the same time, they have the right to conclude on their own additional agreements concerning the non-exclusive distribution of the work in the form in which it was published by this journal, but provided that the link to the first publication of the article in this journal is preserved.
A license agreement is a document in which the author warrants that he/she owns all copyright for the work (manuscript, article, etc.).
The authors, signing the License Agreement with TECHNOLOGY CENTER PC, have all rights to the further use of their work, provided that they link to our edition in which the work was published.
According to the terms of the License Agreement, the Publisher TECHNOLOGY CENTER PC does not take away your copyrights and receives permission from the authors to use and dissemination of the publication through the world's scientific resources (own electronic resources, scientometric databases, repositories, libraries, etc.).
In the absence of a signed License Agreement or in the absence of this agreement of identifiers allowing to identify the identity of the author, the editors have no right to work with the manuscript.
It is important to remember that there is another type of agreement between authors and publishers – when copyright is transferred from the authors to the publisher. In this case, the authors lose ownership of their work and may not use it in any way.