New multifunctional bromine-active polymers: synthesis, properties, and antimicrobial activity
DOI:
https://doi.org/10.15587/1729-4061.2023.278000Keywords:
antimicrobial polymers, immobilization, N-bromamines, N-bromosulfonamides, active chlorine, N-bromotaurine, zone of microbial growth inhibitionAbstract
The increase in the frequency and scale of epidemics of infectious diseases gives extreme urgency to the development of new technologies for antiseptic and disinfectant treatment of various media, as well as materials/reagents for their implementation. Antimicrobial polymer materials of various chemical structures, including those containing halogen-active functional groups, are promising in this regard.
This work is devoted to the synthesis and investigation of the properties of granular and fibrous polymer materials with immobilized N-bromosulfonamide groups of different structure. It is shown that copolymers of styrene with divinylbenzene and polypropylene can be used as a carrier polymer. A technique has been developed that allows obtaining polymers with a content of up to 23 % of immobilized active bromine. The compliance of the synthesized materials with the declared structure has been proven by IR spectroscopy and a complex of chemical methods. A decrease in the strength of the obtained polymers compared to the original carriers has been observed, especially in the case of fibers. The stability of the synthesized polymers during storage is lower that of the previously described chlorine-active analogs. For the quantitative determination of active bromine in the target materials, a technique based on its rapid diffusion from the polymer into the taurine solution has been developed. Microbiological research has shown that the synthesized polymers have a pronounced antimicrobial activity, which is higher than that of immobilized N-chlorosulfonamides and is manifested even in the presence of a significant organic load.
The set of investigated characteristics of synthesized polymers with immobilized N-bromosulfonamide groups suggests the prospect of their use as components of antiseptic dressing materials, antimicrobial filters, devices for obtaining antiseptic solutions, and other medical products
References
- Shang, Y., Li, H., Zhang, R. (2021). Effects of Pandemic Outbreak on Economies: Evidence From Business History Context. Frontiers in Public Health, 9. doi: https://doi.org/10.3389/fpubh.2021.632043
- Kaye, A. D., Okeagu, C. N., Pham, A. D., Silva, R. A., Hurley, J. J., Arron, B. L. et al. (2021). Economic impact of COVID-19 pandemic on healthcare facilities and systems: International perspectives. Best Practice & Research Clinical Anaesthesiology, 35 (3), 293–306. doi: https://doi.org/10.1016/j.bpa.2020.11.009
- Marani, M., Katul, G. G., Pan, W. K., Parolari, A. J. (2021). Intensity and frequency of extreme novel epidemics. Proceedings of the National Academy of Sciences, 118 (35). doi: https://doi.org/10.1073/pnas.2105482118
- Gupta, S., Rouse, B. T., Sarangi, P. P. (2021). Did Climate Change Influence the Emergence, Transmission, and Expression of the COVID-19 Pandemic? Frontiers in Medicine, 8. doi: https://doi.org/10.3389/fmed.2021.769208
- Rodó, X., San-José, A., Kirchgatter, K., López, L. (2021). Changing climate and the COVID-19 pandemic: more than just heads or tails. Nature Medicine, 27 (4), 576–579. doi: https://doi.org/10.1038/s41591-021-01303-y
- Li, R., Richmond, P., Roehner, B. M. (2018). Effect of population density on epidemics. Physica A: Statistical Mechanics and Its Applications, 510, 713–724. doi: https://doi.org/10.1016/j.physa.2018.07.025
- Hollingsworth, T. D., Ferguson, N. M., Anderson, R. M. (2007). Frequent Travelers and Rate of Spread of Epidemics. Emerging Infectious Diseases, 13 (9), 1288–1294. doi: https://doi.org/10.3201/eid1309.070081
- Nikolich-Žugich, J. (2017). The twilight of immunity: emerging concepts in aging of the immune system. Nature Immunology, 19 (1), 10–19. doi: https://doi.org/10.1038/s41590-017-0006-x
- Diani, S., Leonardi, E., Cavezzi, A., Ferrari, S., Iacono, O., Limoli, A. et al. (2022). SARS-CoV-2—The Role of Natural Immunity: A Narrative Review. Journal of Clinical Medicine, 11 (21), 6272. doi: https://doi.org/10.3390/jcm11216272
- Bloom, D. E., Cadarette, D. (2019). Infectious Disease Threats in the Twenty-First Century: Strengthening the Global Response. Frontiers in Immunology, 10. doi: https://doi.org/10.3389/fimmu.2019.00549
- Bai, Y., Wang, Q., Liu, M., Bian, L., Liu, J., Gao, F. et al. (2022). The next major emergent infectious disease: reflections on vaccine emergency development strategies. Expert Review of Vaccines, 21 (4), 471–481. doi: https://doi.org/10.1080/14760584.2022.2027240
- Cozad, A., Jones, R. D. (2003). Disinfection and the prevention of infectious disease. American Journal of Infection Control, 31 (4), 243–254. doi: https://doi.org/10.1067/mic.2003.49
- Prajapati, P., Desai, H., Chandarana, C. (2022). Hand sanitizers as a preventive measure in COVID-19 pandemic, its characteristics, and harmful effects: a review. Journal of the Egyptian Public Health Association, 97 (1). doi: https://doi.org/10.1186/s42506-021-00094-x
- Siddiqui, A. A. (2020). The Role of Personal Protective Equipment (PPE) in Prevention of COVID-19 Novel Corona Virus and Fatalities occur due to Non-availability of the PPE. American Journal of Biomedical Science & Research, 9 (6), 490–499. doi: https://doi.org/10.34297/ajbsr.2020.09.001458
- Block, M. S., Rowan, B. G. (2020). Hypochlorous Acid: A Review. Journal of Oral and Maxillofacial Surgery, 78 (9), 1461–1466. doi: https://doi.org/10.1016/j.joms.2020.06.029
- Chung, I., Ryu, H., Yoon, S.-Y., Ha, J. C. (2022). Health effects of sodium hypochlorite: review of published case reports. Environmental Analysis Health and Toxicology, 37 (1), e2022006. doi: https://doi.org/10.5620/eaht.2022006
- Dong, A., Wang, Y.-J., Gao, Y., Gao, T., Gao, G. (2017). Chemical Insights into Antibacterial N-Halamines. Chemical Reviews, 117 (6), 4806–4862. doi: https://doi.org/10.1021/acs.chemrev.6b00687
- Ashby, L. V., Springer, R., Hampton, M. B., Kettle, A. J., Winterbourn, C. C. (2020). Evaluating the bactericidal action of hypochlorous acid in culture media. Free Radical Biology and Medicine, 159, 119–124. doi: https://doi.org/10.1016/j.freeradbiomed.2020.07.033
- Bernardi, A. O., Stefanello, A., Garcia, M. V., Parussolo, G., Stefanello, R. F., Moro, C. B., Copetti, M. V. (2018). Efficacy of commercial sanitizers against fungi of concern in the food industry. LWT, 97, 25–30. doi: https://doi.org/10.1016/j.lwt.2018.06.037
- Miyaoka, Y., Kabir, Md. H., Hasan, Md. A., Yamaguchi, M., Shoham, D., Murakami, H., Takehara, K. (2021). Virucidal activity of slightly acidic hypochlorous acid water toward influenza virus and coronavirus with tests simulating practical usage. Virus Research, 297, 198383. doi: https://doi.org/10.1016/j.virusres.2021.198383
- Williams, K., Hughson, A. G., Chesebro, B., Race, B. (2019). Inactivation of chronic wasting disease prions using sodium hypochlorite. PLOS ONE, 14(10), e0223659. doi: https://doi.org/10.1371/journal.pone.0223659
- Gessa Sorroche, M., Relimpio López, I., García-Delpech, S., Benítez del Castillo, J. M. (2022). Hypochlorous acid as an antiseptic in the care of patients with suspected COVID-19 infection. Archivos de La Sociedad Española de Oftalmología (English Edition), 97 (2), 77–80. doi: https://doi.org/10.1016/j.oftale.2021.01.010
- Kyriakopoulos, A. M., Grapsa, E., Marcinkiewicz, J., Nagl, M. (2019). Swift Cure of a Chronic Wound Infected With Multiresistant Staphylococcus aureus in an Elderly Patient With Stage 5 Renal Disease. The International Journal of Lower Extremity Wounds, 18 (2), 192–196. doi: https://doi.org/10.1177/1534734619834746
- Dakin, M. H. E. (2014). Pat. No. WO2015063468A1. Anti-inflammatory solution comprising sodium hypochlorite. Available at: https://patents.google.com/patent/WO2015063468A1/en
- Lackner, M., Rössler, A., Volland, A., Stadtmüller, M. N., Müllauer, B., Banki, Z. et al. (2022). N-chlorotaurine is highly active against respiratory viruses including SARS-CoV-2 (COVID-19) in vitro. Emerging Microbes & Infections, 11 (1), 1293–1307. doi: https://doi.org/10.1080/22221751.2022.2065932
- Giarratana, N., Rajan, B., Kamala, K., Mendenhall, M., Reiner, G. (2021). A sprayable Acid-Oxidizing solution containing hypochlorous acid (AOS2020) efficiently and safely inactivates SARS-Cov-2: a new potential solution for upper respiratory tract hygiene. European Archives of Oto-Rhino-Laryngology, 278 (8), 3099–3103. doi: https://doi.org/10.1007/s00405-021-06644-5
- Murashevych, B., Girenko, D., Maslak, H., Stepanskyi, D., Abraimova, O., Netronina, O., Zhminko, P. (2021). Acute inhalation toxicity of aerosolized electrochemically generated solution of sodium hypochlorite. Inhalation Toxicology, 34 (1-2), 1–13. doi: https://doi.org/10.1080/08958378.2021.2013348
- Slaughter, R. J., Watts, M., Vale, J. A., Grieve, J. R., Schep, L. J. (2019). The clinical toxicology of sodium hypochlorite. Clinical Toxicology, 57 (5), 303–311. doi: https://doi.org/10.1080/15563650.2018.1543889
- Gow, C. K., Weinhouse, C., Johnson, G. O., Saunders, K. E. (2022). Stability of Free Available Chlorine Levels in Dilute Sodium Hypochlorite Solutions over a 6-Week Period. Journal of the American Association for Laboratory Animal Science, 61 (2), 181–187. doi: https://doi.org/10.30802/aalas-jaalas-21-000080
- Girenko, D. V., Gyrenko, A. A., Nikolenko, N. V. (2019). Potentiometric Determination of Chlorate Impurities in Hypochlorite Solutions. International Journal of Analytical Chemistry, 2019, 1–7. doi: https://doi.org/10.1155/2019/2360420
- Bianculli, R. H., Mase, J. D., Schulz, M. D. (2020). Antiviral Polymers: Past Approaches and Future Possibilities. Macromolecules, 53 (21), 9158–9186. doi: https://doi.org/10.1021/acs.macromol.0c01273
- Kenawy, E.-R., Worley, S. D., Broughton, R. (2007). The Chemistry and Applications of Antimicrobial Polymers: A State-of-the-Art Review. Biomacromolecules, 8 (5), 1359–1384. doi: https://doi.org/10.1021/bm061150q
- Parham, S., Kharazi, A. Z., Bakhsheshi-Rad, H. R., Kharaziha, M., Ismail, A. F., Sharif, S. et al. (2022). Antimicrobial Synthetic and Natural Polymeric Nanofibers as Wound Dressing: A Review. Advanced Engineering Materials, 24 (6), 2101460. doi: https://doi.org/10.1002/adem.202101460
- Shahid, A., Aslam, B., Muzammil, S., Aslam, N., Shahid, M., Almatroudi, A. et al. (2021). The prospects of antimicrobial coated medical implants. Journal of Applied Biomaterials & Functional Materials, 19, 228080002110403. doi: https://doi.org/10.1177/22808000211040304
- Low, J. L., Kao, P. H.-N., Tambyah, P. A., Koh, G. L. E., Ling, H., Kline, K. A., Cheow, W. S., Leong, S. S. J. (2021). Development of a polymer-based antimicrobial coating for efficacious urinary catheter protection. Biotechnology Notes, 2, 1–10. doi: https://doi.org/10.1016/j.biotno.2020.12.001
- Gulati, R., Sharma, S., Sharma, R. K. (2021). Antimicrobial textile: recent developments and functional perspective. Polymer Bulletin, 79 (8), 5747–5771. doi: https://doi.org/10.1007/s00289-021-03826-3
- Pullangott, G., Kannan, U., S., G., Kiran, D. V., Maliyekkal, S. M. (2021). A comprehensive review on antimicrobial face masks: an emerging weapon in fighting pandemics. RSC Advances, 11 (12), 6544–6576. doi: https://doi.org/10.1039/d0ra10009a
- Carmona-Ribeiro, A. M., Araújo, P. M. (2021). Antimicrobial Polymer−Based Assemblies: A Review. International Journal of Molecular Sciences, 22 (11), 5424. doi: https://doi.org/10.3390/ijms22115424
- Kamaruzzaman, N. F., Tan, L. P., Hamdan, R. H., Choong, S. S., Wong, W. K., Gibson, A. J. et al. (2019). Antimicrobial Polymers: The Potential Replacement of Existing Antibiotics? International Journal of Molecular Sciences, 20 (11), 2747. doi: https://doi.org/10.3390/ijms20112747
- Song, Cvelbar, Strazar, Vossebein, Zille (2019). Chemical, Thermo-Mechanical and Antimicrobial Properties of DBD Plasma Treated Disinfectant-Impregnated Wipes during Storage. Polymers, 11 (11), 1769. doi: https://doi.org/10.3390/polym11111769
- Nemeş, N. S., Ardean, C., Davidescu, C. M., Negrea, A., Ciopec, M., Duţeanu, N. et al. (2022). Antimicrobial Activity of Cellulose Based Materials. Polymers, 14 (4), 735. doi: https://doi.org/10.3390/polym14040735
- Santos, M., Fonseca, A., Mendonça, P., Branco, R., Serra, A., Morais, P., Coelho, J. (2016). Recent Developments in Antimicrobial Polymers: A Review. Materials, 9 (7), 599. doi: https://doi.org/10.3390/ma9070599
- Hui, F., Debiemme-Chouvy, C. (2013). Antimicrobial N-Halamine Polymers and Coatings: A Review of Their Synthesis, Characterization, and Applications. Biomacromolecules, 14 (3), 585–601. doi: https://doi.org/10.1021/bm301980q
- Liang, J., Wu, R., Wang, J.-W., Barnes, K., Worley, S. D., Cho, U. et al. (2006). N-halamine biocidal coatings. Journal of Industrial Microbiology & Biotechnology, 34 (2), 157–163. doi: https://doi.org/10.1007/s10295-006-0181-5
- Emerson, D. W. (1990). Polymer-bound active chlorine: disinfection of water in a flow system. Polymer supported reagents. 5. Industrial & Engineering Chemistry Research, 29 (3), 448–450. doi: https://doi.org/10.1021/ie00099a022
- Bogoczek, R., Kociołek-Balawejder, E. (1989). Studies on a macromolecular dichloroamine — the N,N-dichloro poly(styrene-co-divinylbenzene) sulphonamide. Angewandte Makromolekulare Chemie, 169 (1), 119–135. doi: https://doi.org/10.1002/apmc.1989.051690111
- Farah, S., Aviv, O., Laout, N., Ratner, S., Domb, A. J. (2015). Antimicrobial N-brominated hydantoin and uracil grafted polystyrene beads. Journal of Controlled Release, 216, 18–29. doi: https://doi.org/10.1016/j.jconrel.2015.07.013
- Bogoczek, R., Kociołek-Balawejder, E. (1991). N-bromo-poly(styrene-co-divinylbenzene) sulphonamide metal salts. Synthesis and basic properties. Angewandte Makromolekulare Chemie, 188 (1), 85–96. doi: https://doi.org/10.1002/apmc.1991.051880108
- Li, L., Pu, T., Zhanel, G., Zhao, N., Ens, W., Liu, S. (2012). New Biocide with BothN-Chloramine and Quaternary Ammonium Moieties Exerts Enhanced Bactericidal Activity. Advanced Healthcare Materials, 1 (5), 609–620. doi: https://doi.org/10.1002/adhm.201200018
- Chen, Y., Han, Q. (2011). Designing N-halamine based antibacterial surface on polymers: Fabrication, characterization, and biocidal functions. Applied Surface Science, 257 (14), 6034–6039. doi: https://doi.org/10.1016/j.apsusc.2011.01.115
- Toropin, V., Murashevych, B., Stepanskyi, D., Toropin, M., Kremenchutskiy, H., Burmistrov, K. (2019). New forms of immobilized active chlorine and its potential applications in medicine. Journal of the national academy of medical sciences of Ukraine, 25 (3), 340–352. doi: https://doi.org/10.37621/jnamsu-2019-3-340-352
- Murashevych, B., Koshova, I., Surmasheva, E., Girenko, D., Chuiko, V., Stepanskyi, D. (2022). Broad-purpose antimicrobial chlorine-active polymers: suppression of multidrug-resistant microorganisms and microbial penetration resistance. ScienceRise: Pharmaceutical Science, 5 (39), 64–73. doi: https://doi.org/10.15587/2519-4852.2022.266171
- Murashevych, B., Stepanskyi, D., Toropin, V., Mironenko, A., Maslak, H., Burmistrov, K., Teteriuk, N. (2022). Virucidal properties of new multifunctional fibrous N-halamine-immobilized styrene-divinylbenzene copolymers. Journal of Bioactive and Compatible Polymers, 37 (6), 453–468. doi: https://doi.org/10.1177/08839115221121852
- Dronov, S., Mamchur, V., et al. (2019). New wound dressings with prolonged action. Zaporozhye medical journal, 21 (3), 365–373.
- Murashevych, B., Toropin, V., Stepanskyi, D., Maslak, H., Burmistrov, K., Kotok, V., Kovalenko, V. (2021). Synthesis of new immobilized N-chloro-sulfonamides and release of active chlorine from them. EUREKA: Physics and Engineering, 4, 3–13. doi: https://doi.org/10.21303/2461-4262.2021.001929
- Abo-Farha, S. A., Abdel-Aal, A. Y., Ashour, I. A., Garamon, S. E. (2009). Removal of some heavy metal cations by synthetic resin purolite C100. Journal of Hazardous Materials, 169 (1-3), 190–194. doi: https://doi.org/10.1016/j.jhazmat.2009.03.086
- Soldatov, V. S. (2008). Syntheses and the Main Properties of Fiban Fibrous Ion Exchangers. Solvent Extraction and Ion Exchange, 26 (5), 457–513. doi: https://doi.org/10.1080/07366290802301358
- Walczewska, M., Peruń, A., Białecka, A., Śróttek, M., Jamróz, W., Dorożyński, P. et al. (2017). Comparative Analysis of Microbicidal and Anti-inflammatory Properties of Novel Taurine Bromamine Derivatives and Bromamine T. Taurine 10, 515–534. doi: https://doi.org/10.1007/978-94-024-1079-2_41
- Williams, W. J. (1979). Handbook of Anion Determination. Butterworth-Heinemann. doi: https://doi.org/10.1016/c2013-0-06303-7
- Kurisaki, K., Yoshimura, K. (2007). Kinetics of Sulfate Desorption from Strongly Acidic Cation-exchange Resin. Journal of Ion Exchange, 18 (4), 158–161. doi: https://doi.org/10.5182/jaie.18.158
- Balouiri, M., Sadiki, M., Ibnsouda, S. K. (2016). Methods for in vitro evaluating antimicrobial activity: A review. Journal of Pharmaceutical Analysis, 6 (2), 71–79. doi: https://doi.org/10.1016/j.jpha.2015.11.005
- Qisheng, R., Wenqiang, H. et. al. (1990). Infrared spectrum feature of the sulfonyl group bound to crosslinked polystyrenes. Acta Polymerica Sinica, 1 (1), 7–12.
- Gowda, B. T., Usha, K. M. (2003). Infrared and NMR (1H & 13C) Spectra of Sodium Salts of N-Bromo-Mono and Di-Substituted-Benzenesulphonamides. Zeitschrift Für Naturforschung A, 58 (5-6), 351–356. doi: https://doi.org/10.1515/zna-2003-5-618
- Bogoczek, R., Kociołek-Balawejder, E. (1993). Synthesis and basic characterization of a macromolecular dibromoamine: N,N-dibromo-poly(styrene-co-divinylbenzene)sulfonamide. Polymer, 34 (13), 2883–2888. doi: https://doi.org/10.1016/0032-3861(93)90135-w
- Gottardi, W., Klotz, S., Nagl, M. (2014). Superior bactericidal activity ofN-bromine compounds compared to theirN-chlorine analogues can be reversed under protein load. Journal of Applied Microbiology, 116 (6), 1427–1437. doi: https://doi.org/10.1111/jam.12474
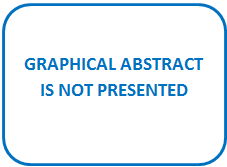
Downloads
Published
How to Cite
Issue
Section
License
Copyright (c) 2023 Bohdan Murashevych, Dmitry Girenko, Mykola Toropin, Iryna Koshova, Vadym Kovalenko, Oleg Lebed, Vasyl Chuiko, Valerii Kotok, Dmytro Stepanskyi

This work is licensed under a Creative Commons Attribution 4.0 International License.
The consolidation and conditions for the transfer of copyright (identification of authorship) is carried out in the License Agreement. In particular, the authors reserve the right to the authorship of their manuscript and transfer the first publication of this work to the journal under the terms of the Creative Commons CC BY license. At the same time, they have the right to conclude on their own additional agreements concerning the non-exclusive distribution of the work in the form in which it was published by this journal, but provided that the link to the first publication of the article in this journal is preserved.
A license agreement is a document in which the author warrants that he/she owns all copyright for the work (manuscript, article, etc.).
The authors, signing the License Agreement with TECHNOLOGY CENTER PC, have all rights to the further use of their work, provided that they link to our edition in which the work was published.
According to the terms of the License Agreement, the Publisher TECHNOLOGY CENTER PC does not take away your copyrights and receives permission from the authors to use and dissemination of the publication through the world's scientific resources (own electronic resources, scientometric databases, repositories, libraries, etc.).
In the absence of a signed License Agreement or in the absence of this agreement of identifiers allowing to identify the identity of the author, the editors have no right to work with the manuscript.
It is important to remember that there is another type of agreement between authors and publishers – when copyright is transferred from the authors to the publisher. In this case, the authors lose ownership of their work and may not use it in any way.