Optimizing the utilization of structural resources of flight simulator motion systems
DOI:
https://doi.org/10.15587/1729-4061.2023.282129Keywords:
power hydraulic drives, dynamic bench, structural resources, aviation simulator, simulation of acceleration actionsAbstract
The object of the study is a six degrees-of-freedom motion system of the synergistic type of a flight simulator. The latter is the main technical means for training pilots and means of research and development of aircraft. The task of optimal utilization of its structural resources was solved, which provides an opportunity to improve the quality of motion cueing. The result is the developed method, which ensures optimal use of structural resources of motion systems of flight simulators. This is explained, first of all, by the use of the developed simplified operator for converting the movements of jacks into the movement of a motion system along individual degrees of freedom on the basis of quadratic approximation. Given this, it became possible to describe the coordinates of the centers of the axes of rotation of the motion system by cubic spline functions. Secondly, the solution of the task of estimating the structural resources of the motion system along linear degrees of freedom on the basis of the developed criterion was carried out by an effective modified method of the deformed polyhedron. This method combines the random search method in the first steps of the search and the gradient method in determining the global extremum. Thirdly, the problem of determining the dependence of the coordinates of the pitch and yaw axes along the pitch angle was stated and solved. Owing to the optimal utilization of the structural resources of motion systems, the coordinates of the axis of their rotation along pitch are as close as possible to the coordinate of the axis of the aircraft. Thus, the quality of motion cueing on the flight simulator is significantly increased and it is possible to use motion systems with shorter lengths of jacks, and therefore, to reduce the cost of their manufacture and operation
References
- Kabanyachyi, V., Sukhov, V. (2022). Sensor calibration of flight simulator motion system. Modern Engineering and Innovative Technologies, 1 (22-01), 127–134. doi: https://doi.org/10.30890/2567-5273.2022-22-01-021
- Andrievskiy, B. R., Arseniev, D. G., Zegzhda, S. A., Kazunin, D. V., Kuznetsov, N. V., Leonov, G. A. et al. (2017). Dynamics of the Stewart platform. Vestnik of Saint Petersburg University. Mathematics. Mechanics. Astronomy, 4 (62 (3)), 489–506. doi: https://doi.org/10.21638/11701/spbu01.2017.311
- Chandrasekaran, K., Theningaledathil, V., Hebbar, A. (2021). Ground based variable stability flight simulator. Aviation, 25 (1), 22–34. doi: https://doi.org/10.3846/aviation.2021.13564
- Markou, A. A., Elmas, S., Filz, G. H. (2021). Revisiting Stewart–Gough platform applications: A kinematic pavilion. Engineering Structures, 249, 113304. doi: https://doi.org/10.1016/j.engstruct.2021.113304
- Hurtasenko, A., Chuev, K., Voloshkin, A., Cherednikov, I., Gavrilov, D. (2022). Optimization of the design parameters of robotic mobility platforms for training machine operators on the simulator and the implementation of the required trajectories. Bulletin of Belgorod State Technological University Named after. V. G. Shukhov, 7 (4), 101–115. doi: https://doi.org/10.34031/2071-7318-2021-7-4-101-115
- Daş, T., Kumpas, I. (2019). Mathematical Modelling, Simulation and Application of Full Flight Helicopter Simulator. Uluslararası Muhendislik Arastirma ve Gelistirme Dergisi, 11 (1), 135–140. doi: https://doi.org/10.29137/umagd.454156
- Virgil Petrescu, R. V., Aversa, R., Apicella, A., Kozaitis, S., Abu-Lebdeh, T., Petrescu, F. I. T. (2018). Inverse Kinematics of a Stewart Platform. Journal of Mechatronics and Robotics, 2 (1), 45–59. doi: https://doi.org/10.3844/jmrsp.2018.45.59
- Sapunov, E. A., Proshin, I. A. (2011). Modeling of the dynamic stand drive at aviation training simulator. Yzvestyia Samarskoho nauchnoho tsentra Rossyiskoi akademiy nauk, 13 (1-2), 337–340.
- Scholten, P. A., van Paassen, M. M., Chu, Q. P., Mulder, M. (2020). Variable Stability In-Flight Simulation System Based on Existing Autopilot Hardware. Journal of Guidance, Control, and Dynamics, 43 (12), 2275–2288. doi: https://doi.org/10.2514/1.g005066
- Silva, D., Garrido, J., Riveiro, E. (2022). Stewart Platform Motion Control Automation with Industrial Resources to Perform Cycloidal and Oceanic Wave Trajectories. Machines, 10 (8), 711. doi: https://doi.org/10.3390/machines10080711
- Velasco, J., Calvo, I., Barambones, O., Venegas, P., Napole, C. (2020). Experimental Validation of a Sliding Mode Control for a Stewart Platform Used in Aerospace Inspection Applications. Mathematics, 8 (11), 2051. doi: https://doi.org/10.3390/math8112051
- Yang, F., Tan, X., Wang, Z., Lu, Z., He, T. (2022). A Geometric Approach for Real-Time Forward Kinematics of the General Stewart Platform. Sensors, 22 (13), 4829. doi: https://doi.org/10.3390/s22134829
- Teodorescu, P. P. (2007). Kinematics. Mathematical and Analytical Techniques with Applications to Engineering, 287–351. doi: https://doi.org/10.1007/1-4020-5442-4_5
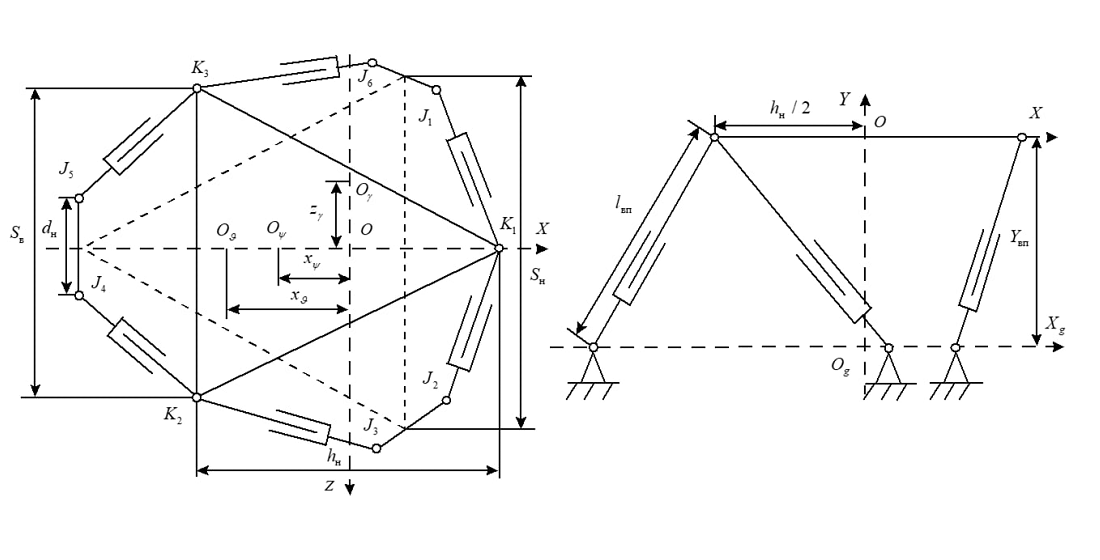
Downloads
Published
How to Cite
Issue
Section
License
Copyright (c) 2023 Volodymyr Kabanyachyi, Serhii Hrytsan

This work is licensed under a Creative Commons Attribution 4.0 International License.
The consolidation and conditions for the transfer of copyright (identification of authorship) is carried out in the License Agreement. In particular, the authors reserve the right to the authorship of their manuscript and transfer the first publication of this work to the journal under the terms of the Creative Commons CC BY license. At the same time, they have the right to conclude on their own additional agreements concerning the non-exclusive distribution of the work in the form in which it was published by this journal, but provided that the link to the first publication of the article in this journal is preserved.
A license agreement is a document in which the author warrants that he/she owns all copyright for the work (manuscript, article, etc.).
The authors, signing the License Agreement with TECHNOLOGY CENTER PC, have all rights to the further use of their work, provided that they link to our edition in which the work was published.
According to the terms of the License Agreement, the Publisher TECHNOLOGY CENTER PC does not take away your copyrights and receives permission from the authors to use and dissemination of the publication through the world's scientific resources (own electronic resources, scientometric databases, repositories, libraries, etc.).
In the absence of a signed License Agreement or in the absence of this agreement of identifiers allowing to identify the identity of the author, the editors have no right to work with the manuscript.
It is important to remember that there is another type of agreement between authors and publishers – when copyright is transferred from the authors to the publisher. In this case, the authors lose ownership of their work and may not use it in any way.