Determination of heat transfer process in vertical cable tunnels of nuclear power plants under real fire conditions
DOI:
https://doi.org/10.15587/1729-4061.2023.289291Keywords:
full-scale fire tests, nuclear power plant, vertical cable tunnel, fire temperature regimeAbstract
The object of the study was heat and mass transfer processes occurring in vertical cable tunnels. The problem to be solved was the definition of process mechanisms in the inner space of the tunnel. For this, field tests were conducted, mathematical models were created, and computational experiments were conducted to establish specific parameters that affect the temperature regime of a fire in a vertical cable tunnel of a nuclear power plant. The dynamics of temperature changes with known geometric parameters and fire load were determined, and the adequacy of mathematical models built in the Fire Dynamics Simulator software was investigated and computational experiments were carried out. It has been proven that they consist in determining the temperature regime in a vertical cable tunnel of a nuclear power plant with known technical and geometric parameters. Such studies have practical applications in the field of safety of nuclear power plants and the development of new technologies in this field. An important conclusion of these studies is the possibility of determining the fire resistance of building structures of vertical cable tunnels of nuclear power plants with the selection of the most severe temperature regime, according to the conducted field test. This means that research results can be used in practice in designing and evaluating the safety of such objects.
The conducted research established that the temperature in the inner space of the tunnel can reach values from 1200 to 1400 ℃. The following factors influence the maximum temperature value and the maximum time to reach the maximum temperature in the fire cell: fire load, height and area of the tunnel. With a lower fire load, the maximum temperature in the vertical cable tunnel of the nuclear power plant was 75 % lower. Therefore, the results of these studies have a direct practical application in the field of safety of nuclear power plants and can be used to improve and develop new technologies in this field
References
- International Atomic Energy Agency. Fire Safety in the Operation of Nuclear Power Plants, IAEA Safety Standards Series No. NS-G-2.1, IAEA (2000). Vienna. Available at: https://www.iaea.org/publications/6018/fire-safety-in-the-operation-of-nuclear-power-plants
- UNE EN 1991-1-2:2019. Eurocode 1: Actions on structures - Part 1-2: General actions - Actions on structures exposed to fire. Available at: https://www.en-standard.eu/une-en-1991-1-2-2019-eurocode-1-actions-on-structures-part-1-2-general-actions-actions-on-structures-exposed-to-fire/?gclid=EAIaIQobChMI7PTbgLL_gQMVRFuRBR08ewG3EAAYASAAEgKBcfD_BwE
- EN 1992-1-2 (2004) (English): Eurocode 2: Design of concrete structures - Part 1-2: General rules - Structural fire design. Available at: https://www.phd.eng.br/wp-content/uploads/2015/12/en.1992.1.2.2004.pdf
- VII.4 Fire resistance of structures. Available at: https://tunnelsmanual.piarc.org/sites/tunnels-manual/files/public/wysiwyg/import/Chapters%20PIARC%20reports/1999%2005.05.B%20Chap%207.4%20EN.pdf
- Kovalyshyn, V. V. (2013). Perevirka na adekvatnist modeliuvannia protsesiv rozvytku i hasinnia pozhezh v kabelnykh tuneliakh (v obmezhenykh obiemakh). Naukovyi visnyk Ukrainskoho naukovo-doslidnoho instytutu pozhezhnoi bezpeky, 1 (27), 38–44.
- Ji, J., Bi, Y., Venkatasubbaiah, K., Li, K. (2016). Influence of aspect ratio of tunnel on smoke temperature distribution under ceiling in near field of fire source. Applied Thermal Engineering, 106, 1094–1102. doi: https://doi.org/10.1016/j.applthermaleng.2016.06.086
- Tian, X., Zhong, M., Shi, C., Zhang, P., Liu, C. (2017). Full-scale tunnel fire experimental study of fire-induced smoke temperature profiles with methanol-gasoline blends. Applied Thermal Engineering, 116, 233–243. doi: https://doi.org/10.1016/j.applthermaleng.2017.01.099
- Modic, J. (2003). Fire simulation in road tunnels. Tunnelling and Underground Space Technology, 18 (5), 525–530. doi: https://doi.org/10.1016/s0886-7798(03)00069-5
- Vaari, J. et al. (2012). Numerical simulations on the performance of waterbased fire suppressions systems. VTT Technology, 54. Available at: https://publications.vtt.fi/pdf/technology/2012/T54.pdf
- Sun, J., Fang, Z., Tang, Z., Beji, T., Merci, B. (2016). Experimental study of the effectiveness of a water system in blocking fire-induced smoke and heat in reduced-scale tunnel tests. Tunnelling and Underground Space Technology, 56, 34–44. doi: https://doi.org/10.1016/j.tust.2016.02.005
- Zhang, P., Tang, X., Tian, X., Liu, C., Zhong, M. (2016). Experimental study on the interaction between fire and water mist in long and narrow spaces. Applied Thermal Engineering, 94, 706–714. doi: https://doi.org/10.1016/j.applthermaleng.2015.10.110
- Troshkin, S. E., Sidney, S. A., Tischenko, E. A., Nekora, O. V. (2015). Issledovanie adekvatnosti rezul'tatov matematicheskogo modelirovaniya dinamiki pozhara v pomeschenii s pomosch'yu programmnogo kompleksa FDS. Pozharnaya bezopasnost': teoriya i praktika, 20, 104–109.
- Forney, G. P. (2007). User‘s Guide for Smokeview Version 5-A Tool for Visualizing Fire Dynamics Simulation Data. NIST Special Publication 1017-1. Available at: https://nvlpubs.nist.gov/nistpubs/SpecialPublications/NIST.SP.1017-1.pdf
- Yelahin, H. I., Yelahin, H. I., Shkarabura, M. H., Kryshtal, M. A., Tyshchenko, O. M. (2013). Osnovy teoriyi rozvytku i prypynennia horinnia. Cherkasy: Akademiya pozhezhnoi bezpeky imeni Heroiv Chornobylia, 460.
- Nuianzin, O. M., Nekora, O. V., Pozdieiev, S. V. et al. (2019). Metody matematychnoho modeliuvannia teplovykh protsesiv pry vyprobuvanniakh na vohnestiykist zalizobetonnykh budivelnykh konstruktsiy. Cherkasy: ChIPB im. Heroiv Chornobylia NUTsZ Ukrainy, 120.
- Kaptsov, I. I. et al. (2009). Metodychni vkazivky do naukovo-doslidnytskoi praktyky z dystsypliny «Orhanizatsiya naukovykh doslidzhen» (Statystychni metody. Analiz ta oformlennia naukovykh doslidzhen). Kharkiv: KhNAMH, 59.
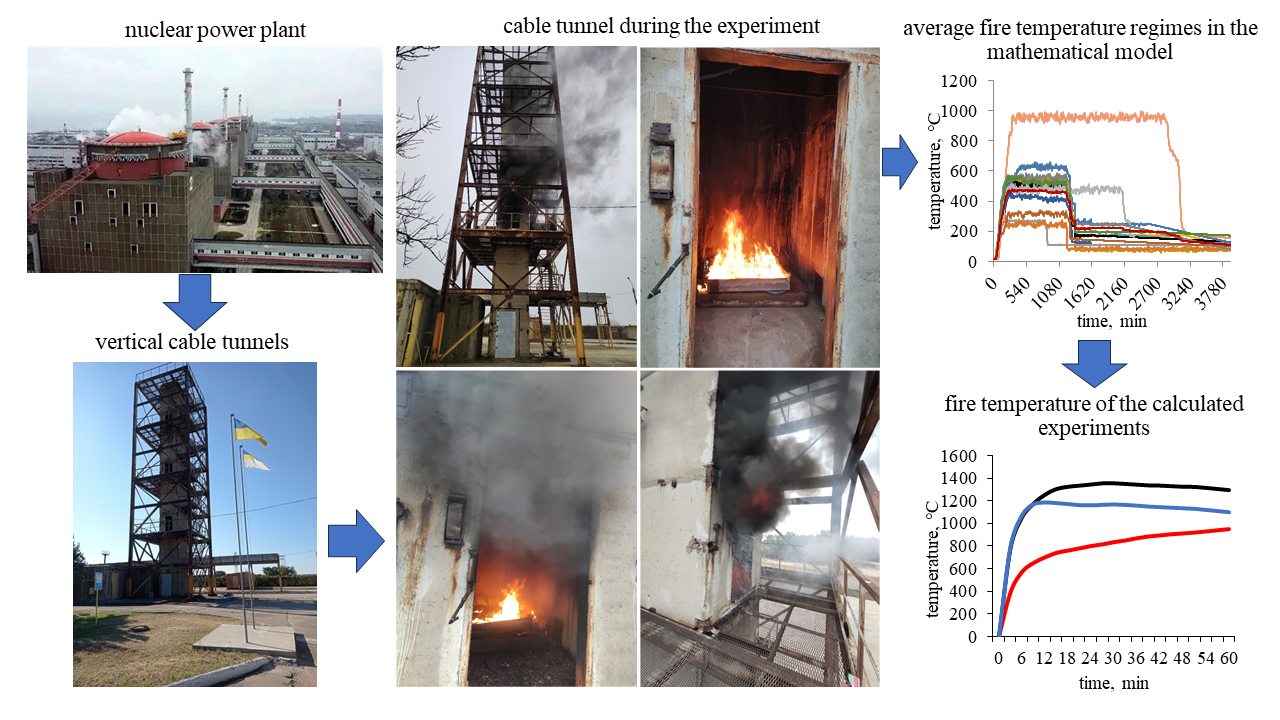
Downloads
Published
How to Cite
Issue
Section
License
Copyright (c) 2023 Serhii Troshkin, Oleh Kulitsa, Serhii Pozdieiev, Tetiana Kostenko, Oleh Zemlianskyi, Nataliia Zaika

This work is licensed under a Creative Commons Attribution 4.0 International License.
The consolidation and conditions for the transfer of copyright (identification of authorship) is carried out in the License Agreement. In particular, the authors reserve the right to the authorship of their manuscript and transfer the first publication of this work to the journal under the terms of the Creative Commons CC BY license. At the same time, they have the right to conclude on their own additional agreements concerning the non-exclusive distribution of the work in the form in which it was published by this journal, but provided that the link to the first publication of the article in this journal is preserved.
A license agreement is a document in which the author warrants that he/she owns all copyright for the work (manuscript, article, etc.).
The authors, signing the License Agreement with TECHNOLOGY CENTER PC, have all rights to the further use of their work, provided that they link to our edition in which the work was published.
According to the terms of the License Agreement, the Publisher TECHNOLOGY CENTER PC does not take away your copyrights and receives permission from the authors to use and dissemination of the publication through the world's scientific resources (own electronic resources, scientometric databases, repositories, libraries, etc.).
In the absence of a signed License Agreement or in the absence of this agreement of identifiers allowing to identify the identity of the author, the editors have no right to work with the manuscript.
It is important to remember that there is another type of agreement between authors and publishers – when copyright is transferred from the authors to the publisher. In this case, the authors lose ownership of their work and may not use it in any way.