Development of Fe-13.8Cr-8.9Mn alloy for steel biomaterials
DOI:
https://doi.org/10.15587/1729-4061.2023.293009Keywords:
plasma nitriding, Fe-13.8Cr-8.9Mn alloy, biomaterials, surface hardness, corrosion resistance, bone implantAbstract
Traumatic, osteoarthritic, tumoral, and congenital bone issues impact human lives and health. The next generation of bone implants is made from biodegradable materials, including Fe-based materials with superior mechanical properties and high biocompatibility. However, efforts to inhibit the risk of inflammation and bacterial infection due to the biological response and corrosion properties of metals are a significant challenge. This study aims to develop biomaterials based on Fe-Cr-Mn alloys to obtain superior physical and mechanical properties through plasma nitriding. Each sample was plasma-nitridated in a vacuum chamber at various temperatures of 250–450 °C for 3 hours at a pressure of 1.8 kPa. Several main tests were performed to investigate the effects of plasma nitriding, such as the chemical compositions of raw material, surface nitrogen contents, phase changes, thickness, hardness, and corrosion. Those parameters were then used to evaluate plasma nitriding's effectiveness, including observing the change in phenomena at each temperature treatment. The results indicated that forming the S phase on the surface of Fe-13.8Cr-8.9Mn alloy is a saturated solution of nitrogen in ɣ-Fe, where the nitrogen content on the surface increases with increasing nitriding temperature. The layer's surface hardness is uniform across its whole thickness, which reduces as the grade of raw material passes through the nitride layer. The highest hardness at a nitriding temperature of 450 °C reached 625.3 VHN. The findings showed that the corrosion rate decreased significantly, reaching the lowest value, 0.0018 mm/year, at a plasma nitriding temperature of 450 °C. Plasma nitriding could enhance the physical and mechanical properties of Fe-Cr-Mn alloy
References
- Bandyopadhyay, A., Mitra, I., Goodman, S. B., Kumar, M., Bose, S. (2023). Improving biocompatibility for next generation of metallic implants. Progress in Materials Science, 133, 101053. doi: https://doi.org/10.1016/j.pmatsci.2022.101053
- Szczęsny, G., Kopec, M., Politis, D. J., Kowalewski, Z. L., Łazarski, A., Szolc, T. (2022). A Review on Biomaterials for Orthopaedic Surgery and Traumatology: From Past to Present. Materials, 15 (10), 3622. doi: https://doi.org/10.3390/ma15103622
- Zhang, L., Yang, G., Johnson, B. N., Jia, X. (2019). Three-dimensional (3D) printed scaffold and material selection for bone repair. Acta Biomaterialia, 84, 16–33. doi: https://doi.org/10.1016/j.actbio.2018.11.039
- Zhao, C., Liu, W., Zhu, M., Wu, C., Zhu, Y. (2022). Bioceramic-based scaffolds with antibacterial function for bone tissue engineering: A review. Bioactive Materials, 18, 383–398. doi: https://doi.org/10.1016/j.bioactmat.2022.02.010
- Ma, H., Feng, C., Chang, J., Wu, C. (2018). 3D-printed bioceramic scaffolds: From bone tissue engineering to tumor therapy. Acta Biomaterialia, 79, 37–59. doi: https://doi.org/10.1016/j.actbio.2018.08.026
- Prestat, M., Thierry, D. (2021). Corrosion of titanium under simulated inflammation conditions: clinical context and in vitro investigations. Acta Biomaterialia, 136, 72–87. doi: https://doi.org/10.1016/j.actbio.2021.10.002
- Armiento, A. R., Hatt, L. P., Sanchez Rosenberg, G., Thompson, K., Stoddart, M. J. (2020). Functional Biomaterials for Bone Regeneration: A Lesson in Complex Biology. Advanced Functional Materials, 30 (44). doi: https://doi.org/10.1002/adfm.201909874
- Wang, X., Rivera‐Bolanos, N., Jiang, B., Ameer, G. A. (2019). Advanced Functional Biomaterials for Stem Cell Delivery in Regenerative Engineering and Medicine. Advanced Functional Materials, 29 (23). doi: https://doi.org/10.1002/adfm.201809009
- Park, J., Lee, S. J., Jung, T. G., Lee, J. H., Kim, W. D., Lee, J. Y., Park, S. A. (2021). Surface modification of a three-dimensional polycaprolactone scaffold by polydopamine, biomineralization, and BMP-2 immobilization for potential bone tissue applications. Colloids and Surfaces B: Biointerfaces, 199, 111528. doi: https://doi.org/10.1016/j.colsurfb.2020.111528
- Wang, X., Xue, J., Ma, B., Wu, J., Chang, J., Gelinsky, M., Wu, C. (2020). Black Bioceramics: Combining Regeneration with Therapy. Advanced Materials, 32 (48). doi: https://doi.org/10.1002/adma.202005140
- Sheng, X., Li, C., Wang, Z., Xu, Y., Sun, Y., Zhang, W. et al. (2023). Advanced applications of strontium-containing biomaterials in bone tissue engineering. Materials Today Bio, 20, 100636. doi: https://doi.org/10.1016/j.mtbio.2023.100636
- Huang, D., Wang, J., Ren, K., Ji, J. (2020). Functionalized biomaterials to combat biofilms. Biomaterials Science, 8 (15), 4052–4066. doi: https://doi.org/10.1039/d0bm00526f
- Wei, H., Cui, J., Lin, K., Xie, J., Wang, X. (2022). Recent advances in smart stimuli-responsive biomaterials for bone therapeutics and regeneration. Bone Research, 10 (1). doi: https://doi.org/10.1038/s41413-021-00180-y
- Lee, S., Lee, J., Byun, H., Kim, S., Joo, J., Park, H. H., shin, H. (2021). Evaluation of the anti-oxidative and ROS scavenging properties of biomaterials coated with epigallocatechin gallate for tissue engineering. Acta Biomaterialia, 124, 166–178. doi: https://doi.org/10.1016/j.actbio.2021.02.005
- Abdalla, S. S. I., Katas, H., Azmi, F., Busra, M. F. M. (2020). Antibacterial and Anti-Biofilm Biosynthesised Silver and Gold Nanoparticles for Medical Applications: Mechanism of Action, Toxicity and Current Status. Current Drug Delivery, 17 (2), 88–100. doi: https://doi.org/10.2174/1567201817666191227094334
- Jia, P., Wang, Z., Zhang, Y., Zhang, D., Gao, W., Su, Y. et al. (2020). Selective sensing of Fe3+ ions in aqueous solution by a biodegradable platform based lanthanide metal organic framework. Spectrochimica Acta Part A: Molecular and Biomolecular Spectroscopy, 230, 118084. doi: https://doi.org/10.1016/j.saa.2020.118084
- Gorejová, R., Haverová, L., Oriňaková, R., Oriňak, A., Oriňak, M. (2018). Recent advancements in Fe-based biodegradable materials for bone repair. Journal of Materials Science, 54 (3), 1913–1947. doi: https://doi.org/10.1007/s10853-018-3011-z
- Carluccio, D., Xu, C., Venezuela, J., Cao, Y., Kent, D., Bermingham, M. et al. (2020). Additively manufactured iron-manganese for biodegradable porous load-bearing bone scaffold applications. Acta Biomaterialia, 103, 346–360. doi: https://doi.org/10.1016/j.actbio.2019.12.018
- Xu, M., Kang, S., Lu, J., Yan, X., Chen, T., Wang, Z. (2020). Properties of a Plasma-Nitrided Coating and a CrNx Coating on the Stainless Steel Bipolar Plate of PEMFC. Coatings, 10 (2), 183. doi: https://doi.org/10.3390/coatings10020183
- Al-Shalawi, F. D., Mohamed Ariff, A. H., Jung, D.-W., Mohd Ariffin, M. K. A., Seng Kim, C. L., Brabazon, D., Al-Osaimi, M. O. (2023). Biomaterials as Implants in the Orthopedic Field for Regenerative Medicine: Metal versus Synthetic Polymers. Polymers, 15 (12), 2601. doi: https://doi.org/10.3390/polym15122601
- Wang, D., Chen, C., Ma, J., Lei, T. (2007). Microstructure of yttric calcium phosphate bioceramic coatings synthesized by laser cladding. Applied Surface Science, 253 (8), 4016–4020. doi: https://doi.org/10.1016/j.apsusc.2006.08.036
- Chien, C. S., Liu, C. W., Kuo, T. Y., Wu, C. C., Hong, T. F. (2016). Bioactivity of fluorapatite/alumina composite coatings deposited on Ti6Al4V substrates by laser cladding. Applied Physics A, 122 (4). doi: https://doi.org/10.1007/s00339-016-9788-1
- De Angelis, F., Sarteur, N., Buonvivere, M., Vadini, M., Šteffl, M., D’Arcangelo, C. (2022). Meta-analytical analysis on components released from resin-based dental materials. Clinical Oral Investigations, 26 (10), 6015–6041. doi: https://doi.org/10.1007/s00784-022-04625-4
- Ritchie, R. O. (2021). Toughening materials: enhancing resistance to fracture. Philosophical Transactions of the Royal Society A: Mathematical, Physical and Engineering Sciences, 379 (2203), 20200437. doi: https://doi.org/10.1098/rsta.2020.0437
- Katti, K. S. (2004). Biomaterials in total joint replacement. Colloids and Surfaces B: Biointerfaces, 39 (3), 133–142. doi: https://doi.org/10.1016/j.colsurfb.2003.12.002
- Yang, K., Ren, Y. (2010). Nickel-free austenitic stainless steels for medical applications. Science and Technology of Advanced Materials, 11 (1), 014105. doi: https://doi.org/10.1088/1468-6996/11/1/014105
- Hryniewicz, T., Rokosz, K., Filippi, M. (2009). Biomaterial Studies on AISI 316L Stainless Steel after Magnetoelectropolishing. Materials, 2 (1), 129–145. doi: https://doi.org/10.3390/ma2010129
- Kraus, T., Moszner, F., Fischerauer, S., Fiedler, M., Martinelli, E., Eichler, J. et al. (2014). Biodegradable Fe-based alloys for use in osteosynthesis: Outcome of an in vivo study after 52weeks. Acta Biomaterialia, 10 (7), 3346–3353. doi: https://doi.org/10.1016/j.actbio.2014.04.007
- Zhang, J., Zhai, B., Gao, J., Li, Z., Zheng, Y., Ma, M. et al. (2022). Plain metallic biomaterials: opportunities and challenges. Regenerative Biomaterials, 10. doi: https://doi.org/10.1093/rb/rbac093
- Shanina, B. D., Gavriljuk, V. G., Berns, H. (2007). High Strength Stainless Austenitic CrMnN steels ‐ Part III: Electronic Properties. Steel Research International, 78 (9), 724–728. doi: https://doi.org/10.1002/srin.200706276
- Lee, T.-H., Ha, H.-Y., Hwang, B., Kim, S.-J., Shin, E. (2012). Effect of Carbon Fraction on Stacking Fault Energy of Austenitic Stainless Steels. Metallurgical and Materials Transactions A, 43 (12), 4455–4459. doi: https://doi.org/10.1007/s11661-012-1423-y
- Ha, H, Y., Lee, T. H., Oh, C. S., Kim, S. J. (2009). Effects of Carbon on the Corrosion Behaviour in Fe-18Cr-10Mn-N-C Stainless Steels. Steel Research International, 80 (7), 488–492. doi: https://doi.org/10.2374/SRI09SP032
- Gavriljuk, V. G., Shanina, B. D., Berns, H. (2008). A physical concept for alloying steels with carbon+nitrogen. Materials Science and Engineering: A, 481–482, 707–712. doi: https://doi.org/10.1016/j.msea.2006.11.186
- Kang, J., Zhang, F. C., Long, X. Y., Yang, Z. N. (2014). Synergistic enhancing effect of N+C alloying on cyclic deformation behaviors in austenitic steel. Materials Science and Engineering: A, 610, 427–435. doi: https://doi.org/10.1016/j.msea.2014.05.052
- Uggowitzer, P. J., Magdowski, R., Speidel, M. O. (1996). High Nitrogen Steels. Nickel Free High Nitrogen Austenitic Steels. ISIJ International, 36 (7), 901–908. doi: https://doi.org/10.2355/isijinternational.36.901
- Talha, M., Ma, Y., Lin, Y., Pan, Y., Kong, X., Sinha, O. P., Behera, C. K. (2019). Corrosion performance of cold deformed austenitic stainless steels for biomedical applications. Corrosion Reviews, 37 (4), 283–306. doi: https://doi.org/10.1515/corrrev-2019-0004
- Gurappa, I. (2002). Characterization of different materials for corrosion resistance under simulated body fluid conditions. Materials Characterization, 49 (1), 73–79. doi: https://doi.org/10.1016/s1044-5803(02)00320-0
- Chua, K., Khan, I., Malhotra, R., Zhu, D. (2021). Additive manufacturing and 3D printing of metallic biomaterials. Engineered Regeneration, 2, 288–299. doi: https://doi.org/10.1016/j.engreg.2021.11.002
- Eliaz, N. (2019). Corrosion of Metallic Biomaterials: A Review. Materials, 12 (3), 407. doi: https://doi.org/10.3390/ma12030407
- Menthe, E., Bulak, A., Olfe, J., Zimmermann, A., Rie, K.-T. (2000). Improvement of the mechanical properties of austenitic stainless steel after plasma nitriding. Surface and Coatings Technology, 133-134, 259–263. doi: https://doi.org/10.1016/s0257-8972(00)00930-0
- Kartikasari, R., Effendy, M. (2021). Surface characterization of Fe–10Al–25Mn alloy for biomaterial applications. Journal of Materials Research and Technology, 15, 409–415. doi: https://doi.org/10.1016/j.jmrt.2021.08.006
- Menthe, E., Rie, K.-T. (1999). Further investigation of the structure and properties of austenitic stainless steel after plasma nitriding. Surface and Coatings Technology, 116–119, 199–204. doi: https://doi.org/10.1016/s0257-8972(99)00085-7
- Behjati, P., Kermanpur, A., Najafizadeh, A., Samaei Baghbadorani, H., Karjalainen, L. P., Jung, J.-G., Lee, Y.-K. (2014). Effect of Nitrogen Content on Grain Refinement and Mechanical Properties of a Reversion-Treated Ni-Free 18Cr-12Mn Austenitic Stainless Steel. Metallurgical and Materials Transactions A, 45 (13), 6317–6328. doi: https://doi.org/10.1007/s11661-014-2595-4
- Adachi, S., Ueda, N. (2012). Formation of S-phase layer on plasma sprayed AISI 316L stainless steel coating by plasma nitriding at low temperature. Thin Solid Films, 523, 11–14. doi: https://doi.org/10.1016/j.tsf.2012.05.062
- Borgioli, F., Galvanetto, E., Bacci, T. (2016). Low temperature nitriding of AISI 300 and 200 series austenitic stainless steels. Vacuum, 127, 51–60. doi: https://doi.org/10.1016/j.vacuum.2016.02.009
- Kim, T., See, C. W., Li, X., Zhu, D. (2020). Orthopedic implants and devices for bone fractures and defects: Past, present and perspective. Engineered Regeneration, 1, 6–18. doi: https://doi.org/10.1016/j.engreg.2020.05.003
- Kumar, C. S., Singh, G., Poddar, S., Varshney, N., Mahto, S. K., Podder, A. S. et al. (2021). High-manganese and nitrogen stabilized austenitic stainless steel (Fe–18Cr–22Mn–0.65N): a material with a bright future for orthopedic implant devices. Biomedical Materials, 16 (6), 065011. doi: https://doi.org/10.1088/1748-605x/ac265e
- Yang, F., Song, R., Li, Y., Sun, T., Wang, K. (2015). Tensile deformation of low density duplex Fe–Mn–Al–C steel. Materials & Design, 76, 32–39. doi: https://doi.org/10.1016/j.matdes.2015.03.043
- Godec, M., Donik, Č., Kocijan, A., Podgornik, B., Skobir Balantič, D. A. (2020). Effect of post-treated low-temperature plasma nitriding on the wear and corrosion resistance of 316L stainless steel manufactured by laser powder-bed fusion. Additive Manufacturing, 32, 101000. doi: https://doi.org/10.1016/j.addma.2019.101000
- Li, X. Y. (2001). Joint Second PrizeLow Temperature Plasma Nitriding of 316 Stainless Steel – Nature of S Phase and Its Thermal Stability. Surface Engineering, 17 (2), 147–152. doi: https://doi.org/10.1179/026708401101517746
- Gontijo, L. C., Machado, R., Miola, E. J., Casteletti, L. C., Alcântara, N. G., Nascente, P. A. P. (2006). Study of the S phase formed on plasma-nitrided AISI 316L stainless steel. Materials Science and Engineering: A, 431 (1-2), 315–321. doi: https://doi.org/10.1016/j.msea.2006.06.023
- Christiansen, T. L., Hummelshøj, T. S., Somers, M. A. J. (2010). Expanded austenite, crystallography and residual stress. Surface Engineering, 26 (4), 242–247. doi: https://doi.org/10.1179/026708410x12506870724316
- Kartikasari, R., Subardi, A., Wijaya, A. E. (2021). Development of Fe-11Al-xMN alloy steel on cryogenic temperatures. Eastern-European Journal of Enterprise Technologies, 5 (12 (113)), 60–68. doi: https://doi.org/10.15587/1729-4061.2021.243236
- Zhou, R., Northwood, D. O., Liu, C. (2020). On nitrogen diffusion during solution treatment in a high nitrogen austenitic stainless steel. Journal of Materials Research and Technology, 9 (2), 2331–2337. doi: https://doi.org/10.1016/j.jmrt.2019.12.064
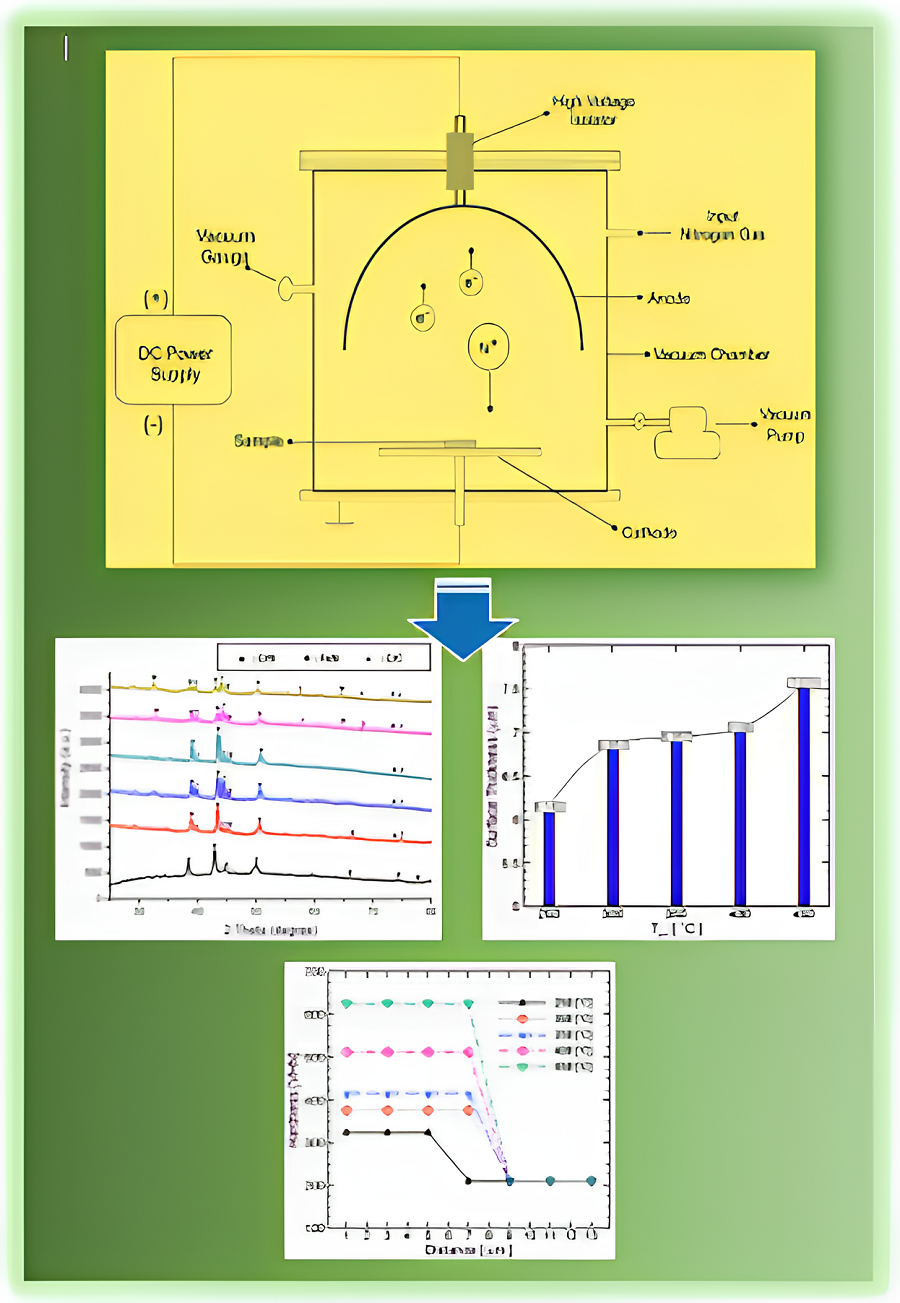
Downloads
Published
How to Cite
Issue
Section
License
Copyright (c) 2023 Ratna Kartikasari, Adi Subardi, Rivan Muhfidin, Ihwanul Aziz, Marwan Effendy, Triyono, Kuncoro Diharjo

This work is licensed under a Creative Commons Attribution 4.0 International License.
The consolidation and conditions for the transfer of copyright (identification of authorship) is carried out in the License Agreement. In particular, the authors reserve the right to the authorship of their manuscript and transfer the first publication of this work to the journal under the terms of the Creative Commons CC BY license. At the same time, they have the right to conclude on their own additional agreements concerning the non-exclusive distribution of the work in the form in which it was published by this journal, but provided that the link to the first publication of the article in this journal is preserved.
A license agreement is a document in which the author warrants that he/she owns all copyright for the work (manuscript, article, etc.).
The authors, signing the License Agreement with TECHNOLOGY CENTER PC, have all rights to the further use of their work, provided that they link to our edition in which the work was published.
According to the terms of the License Agreement, the Publisher TECHNOLOGY CENTER PC does not take away your copyrights and receives permission from the authors to use and dissemination of the publication through the world's scientific resources (own electronic resources, scientometric databases, repositories, libraries, etc.).
In the absence of a signed License Agreement or in the absence of this agreement of identifiers allowing to identify the identity of the author, the editors have no right to work with the manuscript.
It is important to remember that there is another type of agreement between authors and publishers – when copyright is transferred from the authors to the publisher. In this case, the authors lose ownership of their work and may not use it in any way.