Розробка сплаву Fe-13,8Cr-8,9Mn для сталевих біоматеріалів
DOI:
https://doi.org/10.15587/1729-4061.2023.293009Ключові слова:
плазмове азотування, сплав Fe-13,8Cr-8,9Mn, біоматеріали, поверхнева твердість, корозійна стійкість, кістковий імплантатАнотація
Травматичні, остеоартритні, пухлинні та вроджені проблеми з кістками впливають на життя та здоров’я людини. Наступне покоління кісткових імплантатів виготовляється з біорозкладаних матеріалів, включаючи матеріали на основі заліза з чудовими механічними властивостями та високою біосумісністю. Однак спроби зменшити ризик запалення та бактеріальної інфекції через біологічну реакцію та корозійні властивості металів є серйозною проблемою. Це дослідження спрямоване на розробку біоматеріалів на основі сплавів Fe-Cr-Mn для отримання чудових фізико-механічних властивостей шляхом плазмового азотування. Кожен зразок піддавався плазмовому азотуванню у вакуумній камері при різних температурах 250–450 °C протягом 3 годин під тиском 1,8 кПа. Було проведено кілька основних випробувань, щоб дослідити вплив плазмового азотування, наприклад, хімічний склад сировини, вміст азоту на поверхні, фазові зміни, товщину, твердість і корозію. Потім ці параметри використовувалися для оцінки ефективності плазмового азотування, включаючи спостереження за зміною явищ при кожній температурній обробці. Результати показали, що формування S-фази на поверхні сплаву Fe-13,8Cr-8,9Mn є насиченим розчином азоту в ɣ-Fe, де вміст азоту на поверхні збільшується зі збільшенням температури азотування. Твердість поверхні шару рівномірна по всій його товщині, яка зменшується, коли сорт сировини проходить через нітридний шар. Найбільша твердість при температурі азотування 450 °С досягала 625,3 ВГН. Результати показали, що швидкість корозії значно зменшилася, досягнувши найнижчого значення, 0,0018 мм/рік, при температурі плазмового азотування 450 °C. Плазмове азотування може покращити фізико-механічні властивості сплаву Fe-Cr-Mn
Посилання
- Bandyopadhyay, A., Mitra, I., Goodman, S. B., Kumar, M., Bose, S. (2023). Improving biocompatibility for next generation of metallic implants. Progress in Materials Science, 133, 101053. doi: https://doi.org/10.1016/j.pmatsci.2022.101053
- Szczęsny, G., Kopec, M., Politis, D. J., Kowalewski, Z. L., Łazarski, A., Szolc, T. (2022). A Review on Biomaterials for Orthopaedic Surgery and Traumatology: From Past to Present. Materials, 15 (10), 3622. doi: https://doi.org/10.3390/ma15103622
- Zhang, L., Yang, G., Johnson, B. N., Jia, X. (2019). Three-dimensional (3D) printed scaffold and material selection for bone repair. Acta Biomaterialia, 84, 16–33. doi: https://doi.org/10.1016/j.actbio.2018.11.039
- Zhao, C., Liu, W., Zhu, M., Wu, C., Zhu, Y. (2022). Bioceramic-based scaffolds with antibacterial function for bone tissue engineering: A review. Bioactive Materials, 18, 383–398. doi: https://doi.org/10.1016/j.bioactmat.2022.02.010
- Ma, H., Feng, C., Chang, J., Wu, C. (2018). 3D-printed bioceramic scaffolds: From bone tissue engineering to tumor therapy. Acta Biomaterialia, 79, 37–59. doi: https://doi.org/10.1016/j.actbio.2018.08.026
- Prestat, M., Thierry, D. (2021). Corrosion of titanium under simulated inflammation conditions: clinical context and in vitro investigations. Acta Biomaterialia, 136, 72–87. doi: https://doi.org/10.1016/j.actbio.2021.10.002
- Armiento, A. R., Hatt, L. P., Sanchez Rosenberg, G., Thompson, K., Stoddart, M. J. (2020). Functional Biomaterials for Bone Regeneration: A Lesson in Complex Biology. Advanced Functional Materials, 30 (44). doi: https://doi.org/10.1002/adfm.201909874
- Wang, X., Rivera‐Bolanos, N., Jiang, B., Ameer, G. A. (2019). Advanced Functional Biomaterials for Stem Cell Delivery in Regenerative Engineering and Medicine. Advanced Functional Materials, 29 (23). doi: https://doi.org/10.1002/adfm.201809009
- Park, J., Lee, S. J., Jung, T. G., Lee, J. H., Kim, W. D., Lee, J. Y., Park, S. A. (2021). Surface modification of a three-dimensional polycaprolactone scaffold by polydopamine, biomineralization, and BMP-2 immobilization for potential bone tissue applications. Colloids and Surfaces B: Biointerfaces, 199, 111528. doi: https://doi.org/10.1016/j.colsurfb.2020.111528
- Wang, X., Xue, J., Ma, B., Wu, J., Chang, J., Gelinsky, M., Wu, C. (2020). Black Bioceramics: Combining Regeneration with Therapy. Advanced Materials, 32 (48). doi: https://doi.org/10.1002/adma.202005140
- Sheng, X., Li, C., Wang, Z., Xu, Y., Sun, Y., Zhang, W. et al. (2023). Advanced applications of strontium-containing biomaterials in bone tissue engineering. Materials Today Bio, 20, 100636. doi: https://doi.org/10.1016/j.mtbio.2023.100636
- Huang, D., Wang, J., Ren, K., Ji, J. (2020). Functionalized biomaterials to combat biofilms. Biomaterials Science, 8 (15), 4052–4066. doi: https://doi.org/10.1039/d0bm00526f
- Wei, H., Cui, J., Lin, K., Xie, J., Wang, X. (2022). Recent advances in smart stimuli-responsive biomaterials for bone therapeutics and regeneration. Bone Research, 10 (1). doi: https://doi.org/10.1038/s41413-021-00180-y
- Lee, S., Lee, J., Byun, H., Kim, S., Joo, J., Park, H. H., shin, H. (2021). Evaluation of the anti-oxidative and ROS scavenging properties of biomaterials coated with epigallocatechin gallate for tissue engineering. Acta Biomaterialia, 124, 166–178. doi: https://doi.org/10.1016/j.actbio.2021.02.005
- Abdalla, S. S. I., Katas, H., Azmi, F., Busra, M. F. M. (2020). Antibacterial and Anti-Biofilm Biosynthesised Silver and Gold Nanoparticles for Medical Applications: Mechanism of Action, Toxicity and Current Status. Current Drug Delivery, 17 (2), 88–100. doi: https://doi.org/10.2174/1567201817666191227094334
- Jia, P., Wang, Z., Zhang, Y., Zhang, D., Gao, W., Su, Y. et al. (2020). Selective sensing of Fe3+ ions in aqueous solution by a biodegradable platform based lanthanide metal organic framework. Spectrochimica Acta Part A: Molecular and Biomolecular Spectroscopy, 230, 118084. doi: https://doi.org/10.1016/j.saa.2020.118084
- Gorejová, R., Haverová, L., Oriňaková, R., Oriňak, A., Oriňak, M. (2018). Recent advancements in Fe-based biodegradable materials for bone repair. Journal of Materials Science, 54 (3), 1913–1947. doi: https://doi.org/10.1007/s10853-018-3011-z
- Carluccio, D., Xu, C., Venezuela, J., Cao, Y., Kent, D., Bermingham, M. et al. (2020). Additively manufactured iron-manganese for biodegradable porous load-bearing bone scaffold applications. Acta Biomaterialia, 103, 346–360. doi: https://doi.org/10.1016/j.actbio.2019.12.018
- Xu, M., Kang, S., Lu, J., Yan, X., Chen, T., Wang, Z. (2020). Properties of a Plasma-Nitrided Coating and a CrNx Coating on the Stainless Steel Bipolar Plate of PEMFC. Coatings, 10 (2), 183. doi: https://doi.org/10.3390/coatings10020183
- Al-Shalawi, F. D., Mohamed Ariff, A. H., Jung, D.-W., Mohd Ariffin, M. K. A., Seng Kim, C. L., Brabazon, D., Al-Osaimi, M. O. (2023). Biomaterials as Implants in the Orthopedic Field for Regenerative Medicine: Metal versus Synthetic Polymers. Polymers, 15 (12), 2601. doi: https://doi.org/10.3390/polym15122601
- Wang, D., Chen, C., Ma, J., Lei, T. (2007). Microstructure of yttric calcium phosphate bioceramic coatings synthesized by laser cladding. Applied Surface Science, 253 (8), 4016–4020. doi: https://doi.org/10.1016/j.apsusc.2006.08.036
- Chien, C. S., Liu, C. W., Kuo, T. Y., Wu, C. C., Hong, T. F. (2016). Bioactivity of fluorapatite/alumina composite coatings deposited on Ti6Al4V substrates by laser cladding. Applied Physics A, 122 (4). doi: https://doi.org/10.1007/s00339-016-9788-1
- De Angelis, F., Sarteur, N., Buonvivere, M., Vadini, M., Šteffl, M., D’Arcangelo, C. (2022). Meta-analytical analysis on components released from resin-based dental materials. Clinical Oral Investigations, 26 (10), 6015–6041. doi: https://doi.org/10.1007/s00784-022-04625-4
- Ritchie, R. O. (2021). Toughening materials: enhancing resistance to fracture. Philosophical Transactions of the Royal Society A: Mathematical, Physical and Engineering Sciences, 379 (2203), 20200437. doi: https://doi.org/10.1098/rsta.2020.0437
- Katti, K. S. (2004). Biomaterials in total joint replacement. Colloids and Surfaces B: Biointerfaces, 39 (3), 133–142. doi: https://doi.org/10.1016/j.colsurfb.2003.12.002
- Yang, K., Ren, Y. (2010). Nickel-free austenitic stainless steels for medical applications. Science and Technology of Advanced Materials, 11 (1), 014105. doi: https://doi.org/10.1088/1468-6996/11/1/014105
- Hryniewicz, T., Rokosz, K., Filippi, M. (2009). Biomaterial Studies on AISI 316L Stainless Steel after Magnetoelectropolishing. Materials, 2 (1), 129–145. doi: https://doi.org/10.3390/ma2010129
- Kraus, T., Moszner, F., Fischerauer, S., Fiedler, M., Martinelli, E., Eichler, J. et al. (2014). Biodegradable Fe-based alloys for use in osteosynthesis: Outcome of an in vivo study after 52weeks. Acta Biomaterialia, 10 (7), 3346–3353. doi: https://doi.org/10.1016/j.actbio.2014.04.007
- Zhang, J., Zhai, B., Gao, J., Li, Z., Zheng, Y., Ma, M. et al. (2022). Plain metallic biomaterials: opportunities and challenges. Regenerative Biomaterials, 10. doi: https://doi.org/10.1093/rb/rbac093
- Shanina, B. D., Gavriljuk, V. G., Berns, H. (2007). High Strength Stainless Austenitic CrMnN steels ‐ Part III: Electronic Properties. Steel Research International, 78 (9), 724–728. doi: https://doi.org/10.1002/srin.200706276
- Lee, T.-H., Ha, H.-Y., Hwang, B., Kim, S.-J., Shin, E. (2012). Effect of Carbon Fraction on Stacking Fault Energy of Austenitic Stainless Steels. Metallurgical and Materials Transactions A, 43 (12), 4455–4459. doi: https://doi.org/10.1007/s11661-012-1423-y
- Ha, H, Y., Lee, T. H., Oh, C. S., Kim, S. J. (2009). Effects of Carbon on the Corrosion Behaviour in Fe-18Cr-10Mn-N-C Stainless Steels. Steel Research International, 80 (7), 488–492. doi: https://doi.org/10.2374/SRI09SP032
- Gavriljuk, V. G., Shanina, B. D., Berns, H. (2008). A physical concept for alloying steels with carbon+nitrogen. Materials Science and Engineering: A, 481–482, 707–712. doi: https://doi.org/10.1016/j.msea.2006.11.186
- Kang, J., Zhang, F. C., Long, X. Y., Yang, Z. N. (2014). Synergistic enhancing effect of N+C alloying on cyclic deformation behaviors in austenitic steel. Materials Science and Engineering: A, 610, 427–435. doi: https://doi.org/10.1016/j.msea.2014.05.052
- Uggowitzer, P. J., Magdowski, R., Speidel, M. O. (1996). High Nitrogen Steels. Nickel Free High Nitrogen Austenitic Steels. ISIJ International, 36 (7), 901–908. doi: https://doi.org/10.2355/isijinternational.36.901
- Talha, M., Ma, Y., Lin, Y., Pan, Y., Kong, X., Sinha, O. P., Behera, C. K. (2019). Corrosion performance of cold deformed austenitic stainless steels for biomedical applications. Corrosion Reviews, 37 (4), 283–306. doi: https://doi.org/10.1515/corrrev-2019-0004
- Gurappa, I. (2002). Characterization of different materials for corrosion resistance under simulated body fluid conditions. Materials Characterization, 49 (1), 73–79. doi: https://doi.org/10.1016/s1044-5803(02)00320-0
- Chua, K., Khan, I., Malhotra, R., Zhu, D. (2021). Additive manufacturing and 3D printing of metallic biomaterials. Engineered Regeneration, 2, 288–299. doi: https://doi.org/10.1016/j.engreg.2021.11.002
- Eliaz, N. (2019). Corrosion of Metallic Biomaterials: A Review. Materials, 12 (3), 407. doi: https://doi.org/10.3390/ma12030407
- Menthe, E., Bulak, A., Olfe, J., Zimmermann, A., Rie, K.-T. (2000). Improvement of the mechanical properties of austenitic stainless steel after plasma nitriding. Surface and Coatings Technology, 133-134, 259–263. doi: https://doi.org/10.1016/s0257-8972(00)00930-0
- Kartikasari, R., Effendy, M. (2021). Surface characterization of Fe–10Al–25Mn alloy for biomaterial applications. Journal of Materials Research and Technology, 15, 409–415. doi: https://doi.org/10.1016/j.jmrt.2021.08.006
- Menthe, E., Rie, K.-T. (1999). Further investigation of the structure and properties of austenitic stainless steel after plasma nitriding. Surface and Coatings Technology, 116–119, 199–204. doi: https://doi.org/10.1016/s0257-8972(99)00085-7
- Behjati, P., Kermanpur, A., Najafizadeh, A., Samaei Baghbadorani, H., Karjalainen, L. P., Jung, J.-G., Lee, Y.-K. (2014). Effect of Nitrogen Content on Grain Refinement and Mechanical Properties of a Reversion-Treated Ni-Free 18Cr-12Mn Austenitic Stainless Steel. Metallurgical and Materials Transactions A, 45 (13), 6317–6328. doi: https://doi.org/10.1007/s11661-014-2595-4
- Adachi, S., Ueda, N. (2012). Formation of S-phase layer on plasma sprayed AISI 316L stainless steel coating by plasma nitriding at low temperature. Thin Solid Films, 523, 11–14. doi: https://doi.org/10.1016/j.tsf.2012.05.062
- Borgioli, F., Galvanetto, E., Bacci, T. (2016). Low temperature nitriding of AISI 300 and 200 series austenitic stainless steels. Vacuum, 127, 51–60. doi: https://doi.org/10.1016/j.vacuum.2016.02.009
- Kim, T., See, C. W., Li, X., Zhu, D. (2020). Orthopedic implants and devices for bone fractures and defects: Past, present and perspective. Engineered Regeneration, 1, 6–18. doi: https://doi.org/10.1016/j.engreg.2020.05.003
- Kumar, C. S., Singh, G., Poddar, S., Varshney, N., Mahto, S. K., Podder, A. S. et al. (2021). High-manganese and nitrogen stabilized austenitic stainless steel (Fe–18Cr–22Mn–0.65N): a material with a bright future for orthopedic implant devices. Biomedical Materials, 16 (6), 065011. doi: https://doi.org/10.1088/1748-605x/ac265e
- Yang, F., Song, R., Li, Y., Sun, T., Wang, K. (2015). Tensile deformation of low density duplex Fe–Mn–Al–C steel. Materials & Design, 76, 32–39. doi: https://doi.org/10.1016/j.matdes.2015.03.043
- Godec, M., Donik, Č., Kocijan, A., Podgornik, B., Skobir Balantič, D. A. (2020). Effect of post-treated low-temperature plasma nitriding on the wear and corrosion resistance of 316L stainless steel manufactured by laser powder-bed fusion. Additive Manufacturing, 32, 101000. doi: https://doi.org/10.1016/j.addma.2019.101000
- Li, X. Y. (2001). Joint Second PrizeLow Temperature Plasma Nitriding of 316 Stainless Steel – Nature of S Phase and Its Thermal Stability. Surface Engineering, 17 (2), 147–152. doi: https://doi.org/10.1179/026708401101517746
- Gontijo, L. C., Machado, R., Miola, E. J., Casteletti, L. C., Alcântara, N. G., Nascente, P. A. P. (2006). Study of the S phase formed on plasma-nitrided AISI 316L stainless steel. Materials Science and Engineering: A, 431 (1-2), 315–321. doi: https://doi.org/10.1016/j.msea.2006.06.023
- Christiansen, T. L., Hummelshøj, T. S., Somers, M. A. J. (2010). Expanded austenite, crystallography and residual stress. Surface Engineering, 26 (4), 242–247. doi: https://doi.org/10.1179/026708410x12506870724316
- Kartikasari, R., Subardi, A., Wijaya, A. E. (2021). Development of Fe-11Al-xMN alloy steel on cryogenic temperatures. Eastern-European Journal of Enterprise Technologies, 5 (12 (113)), 60–68. doi: https://doi.org/10.15587/1729-4061.2021.243236
- Zhou, R., Northwood, D. O., Liu, C. (2020). On nitrogen diffusion during solution treatment in a high nitrogen austenitic stainless steel. Journal of Materials Research and Technology, 9 (2), 2331–2337. doi: https://doi.org/10.1016/j.jmrt.2019.12.064
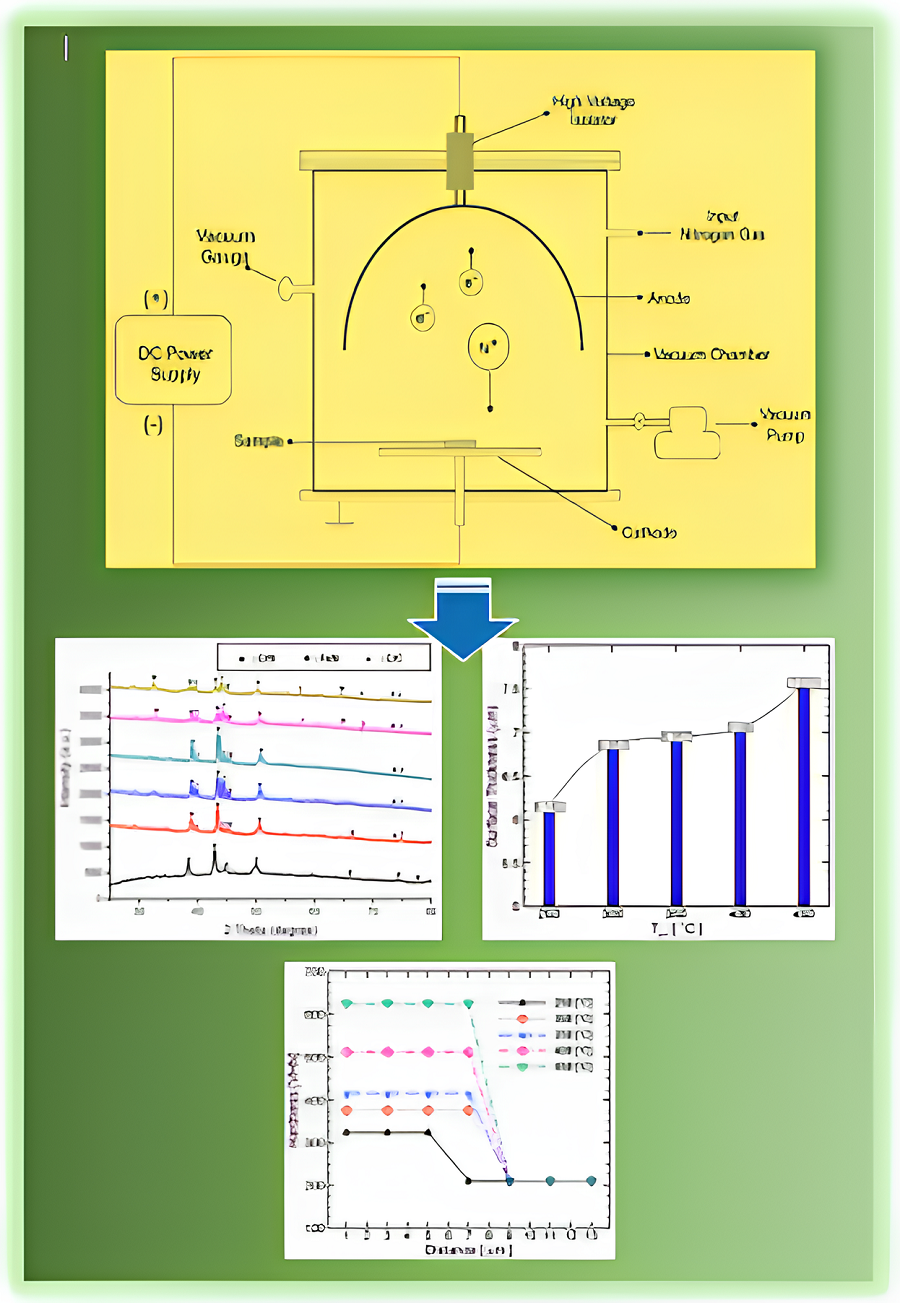
##submission.downloads##
Опубліковано
Як цитувати
Номер
Розділ
Ліцензія
Авторське право (c) 2023 Ratna Kartikasari, Adi Subardi, Rivan Muhfidin, Ihwanul Aziz, Marwan Effendy, Triyono, Kuncoro Diharjo

Ця робота ліцензується відповідно до Creative Commons Attribution 4.0 International License.
Закріплення та умови передачі авторських прав (ідентифікація авторства) здійснюється у Ліцензійному договорі. Зокрема, автори залишають за собою право на авторство свого рукопису та передають журналу право першої публікації цієї роботи на умовах ліцензії Creative Commons CC BY. При цьому вони мають право укладати самостійно додаткові угоди, що стосуються неексклюзивного поширення роботи у тому вигляді, в якому вона була опублікована цим журналом, але за умови збереження посилання на першу публікацію статті в цьому журналі.
Ліцензійний договір – це документ, в якому автор гарантує, що володіє усіма авторськими правами на твір (рукопис, статтю, тощо).
Автори, підписуючи Ліцензійний договір з ПП «ТЕХНОЛОГІЧНИЙ ЦЕНТР», мають усі права на подальше використання свого твору за умови посилання на наше видання, в якому твір опублікований. Відповідно до умов Ліцензійного договору, Видавець ПП «ТЕХНОЛОГІЧНИЙ ЦЕНТР» не забирає ваші авторські права та отримує від авторів дозвіл на використання та розповсюдження публікації через світові наукові ресурси (власні електронні ресурси, наукометричні бази даних, репозитарії, бібліотеки тощо).
За відсутності підписаного Ліцензійного договору або за відсутністю вказаних в цьому договорі ідентифікаторів, що дають змогу ідентифікувати особу автора, редакція не має права працювати з рукописом.
Важливо пам’ятати, що існує і інший тип угоди між авторами та видавцями – коли авторські права передаються від авторів до видавця. В такому разі автори втрачають права власності на свій твір та не можуть його використовувати в будь-який спосіб.