Evaluation of the operational curve for heat storage technology using n-octacosane/low-density as a binding material
DOI:
https://doi.org/10.15587/1729-4061.2024.302627Keywords:
binding material, n-octacosane, operational energy, phase transition, energy storageAbstract
Heat storage technology has a critical role for a number of applications involving renewable thermal energy (such as solar water heater). The application of wax (n-octacosane/OCT) as a medium for storing heat brings many positive influences for the system. However, the operational curve is undesirable for the OCT-based energy storage, which makes it necessary to use a binding material. The present work employed LD-class polymer (LDPE) and linear-LDPE as binding materials for OCT. The mixture is prepared through mechanical hot stirring, which comes into two categories: SOCT1 (OCT/LD) and SOCT2 (OCT/LLD). The assessment through the calorimetry method shows an increment in transition temperature for SOCT with a value of 2.1 °C and 5.5 °C. This contributes to the variation of fusion energy for solid-liquid change for both materials, which amounted to 132.05 J/g (SOCT1) and 113.4 J/g (SOCT2). Another assessment related to its chemical and structural phase demonstrates that SOCT has an identical structure to OCT, indicating that SOCT is mixed physically. At the operational level, SOCT is more optimal than OCT according to the indicator related to charge and discharge duration for energy exchange. SOCT1 demonstrates a short plateau line as the indication of a steady transition between 65.4–67.9 °C, while SOCT2 indicates the average heating rate, which is higher than for single OCT. The heat releasing curve for SOCT1 varies at a lower value between 1.92 °C/min and 0.77 °C/min, while SOCT2 has the lowest variation, which is only 0.17 °C/min. Moreover, the self-insulation for SOCT2 has the lowest rate, which is only 0.3 °C/min. The evaluation and analysis from this work show that SOCT is reliable to increase the operational curve of OCT and can be implemented for thermal systems
References
- Mohammad Firman, L. O., Adji, R. B., Ismail, Rahman, R. A. (2023). Increasing the feasibility and storage property of cellulose-based biomass by forming shape-stabilized briquette with hydrophobic compound. Case Studies in Chemical and Environmental Engineering, 8, 100443. https://doi.org/10.1016/j.cscee.2023.100443
- Khademi, A., Darbandi, M., Schneider, G. E. (2020). Numerical Study to Optimize the Melting Process of Phase Change Material Coupled with Extra Fluid. AIAA Scitech 2020 Forum. https://doi.org/10.2514/6.2020-1932
- Suyitno, B. M., Rahman, R. A., Sukma, H., Rahmalina, D. (2022). The assessment of reflector material durability for concentrated solar power based on environment exposure and accelerated aging test. Eastern-European Journal of Enterprise Technologies, 6 (12 (120)), 22–29. https://doi.org/10.15587/1729-4061.2022.265678
- Favakeh, A., Khademi, A., Shafii, M. B. (2019). Experimental study of double solid phase change material in a cavity. 7th International Conference on Energy Research and Development, ICERD 2019, 24–31.
- Abtahi Mehrjardi, S. A., Khademi, A., Fazli, M. (2024). Optimization of a thermal energy storage system enhanced with fins using generative adversarial networks method. Thermal Science and Engineering Progress, 49, 102471. https://doi.org/10.1016/j.tsep.2024.102471
- Khademi, A., Mehrjardi, S. A. A., Said, Z., Chamkha, A. J. (2023). Heat Transfer Improvement in a Thermal Energy Storage System using Auxiliary Fluid Instead of Nano-PCM in an Inclined Enclosure: A Comparative Study. Journal of Applied and Computational Mechanics, 9 (2), 475–486. https://doi.org/10.22055/jacm.2022.41867.3829
- Zariatin, D. L., Suwandi, A. (2023). Advanced design of a small-scale mini gerotor pump in a high-temperature and high-viscosity fluid thermal system. Eastern-European Journal of Enterprise Technologies, 1 (8 (121)), 30–39. https://doi.org/10.15587/1729-4061.2023.272674
- Khademi, A., Darbandi, M., Behshad Shafii, M., Schneider, G. (2019). Numerical Simulation of Phase Change Materials to Predict the Energy Storage Process Accurately. AIAA Propulsion and Energy 2019 Forum. https://doi.org/10.2514/6.2019-4225
- Khademi, A., Mehrjardi, S. A. A., Tiari, S., Mazaheri, K., Shafii, M. B. (2022). Thermal Efficiency Improvement of Brayton Cycle in the Presence of Phase Change Material. Proceedings of the 9th International Conference on Fluid Flow, Heat and Mass Transfer (FFHMT’22). https://doi.org/10.11159/ffhmt22.135
- Favakeh, A., Khademi, A., Shafii, M. B. (2022). Experimental Investigation of the Melting Process of Immiscible Binary Phase Change Materials. Heat Transfer Engineering, 44 (2), 154–174. https://doi.org/10.1080/01457632.2022.2034085
- Rathore, P. K. S., Shukla, S. kumar. (2021). Improvement in thermal properties of PCM/Expanded vermiculite/expanded graphite shape stabilized composite PCM for building energy applications. Renewable Energy, 176, 295–304. https://doi.org/10.1016/j.renene.2021.05.068
- He, M., Xie, D., Yin, L., Gong, K., Zhou, K. (2023). Influences of reduction temperature on energy storage performance of paraffin wax/graphene aerogel composite phase change materials. Materials Today Communications, 34, 105288. https://doi.org/10.1016/j.mtcomm.2022.105288
- Li, S., Wang, H., Gao, X., Niu, Z., Song, J. (2023). Design of corn straw/paraffin wax shape-stabilized phase change materials with excellent thermal buffering performance. Journal of Energy Storage, 57, 106217. https://doi.org/10.1016/j.est.2022.106217
- Zarrinjooy Alvar, M., Abdeali, G., Bahramian, A. R. (2022). Influence of graphite nano powder on ethylene propylene diene monomer/paraffin wax phase change material composite: Shape stability and thermal applications. Journal of Energy Storage, 52, 105065. https://doi.org/10.1016/j.est.2022.105065
- Rahmalina, D., Zada, A. R., Soefihandini, H., Ismail, I., Suyitno, B. M. (2023). Analysis of the thermal characteristics of the paraffin wax/high-density polyethylene (HDPE) composite as a form-stable phase change material (FSPCM) for thermal energy storage. Eastern-European Journal of Enterprise Technologies, 1 (6 (121)), 6–13. https://doi.org/10.15587/1729-4061.2023.273437
- Sciacovelli, A., Navarro, M. E., Jin, Y., Qiao, G., Zheng, L., Leng, G. et al. (2018). High density polyethylene (HDPE) – Graphite composite manufactured by extrusion: A novel way to fabricate phase change materials for thermal energy storage. Particuology, 40, 131–140. https://doi.org/10.1016/j.partic.2017.11.011
- Abtahi Mehrjardi, S. A., Khademi, A., Said, Z., Ushak, S., Chamkha, A. J. (2023). Enhancing latent heat storage systems: The impact of PCM volumetric ratios on energy storage rates with auxiliary fluid assistance. Energy Nexus, 11, 100227. https://doi.org/10.1016/j.nexus.2023.100227
- Wang, H., Rao, Z., Li, L., Liao, S. (2023). A novel composite phase change material of high-density polyethylene/d-mannitol/expanded graphite for medium-temperature thermal energy storage: Characterization and thermal properties. Journal of Energy Storage, 60, 106603. https://doi.org/10.1016/j.est.2022.106603
- Harmen, Y., Chhiti, Y., Alaoui, F. E. M., Bentiss, F., Elkhouakhi, M., Deshayes, L. et al. (2020). Storage efficiency of paraffin-LDPE-MWCNT phase change material for industrial building applications. 2020 5th International Conference on Renewable Energies for Developing Countries (REDEC). https://doi.org/10.1109/redec49234.2020.9163856
- Yu, Y., Qin, H., Ran, S., Song, J., Xia, W., Wang, S., Xiong, C. (2023). A Low-Density Polyethylene-Reinforced Ternary Phase-Change Composite with High Thermal Conductivity for Battery Thermal Management. Energies, 16 (9), 3838. https://doi.org/10.3390/en16093838
- Al-Gunaid, T., Sobolčiak, P., Chriaa, I., Karkri, M., Mrlik, M., Ilčíková, M. et al. (2023). Phase change materials designed from Tetra Pak waste and paraffin wax as unique thermal energy storage systems. Journal of Energy Storage, 64, 107173. https://doi.org/10.1016/j.est.2023.107173
- Zhao, J., Rui, Z., Hu, Z., Shangguan, Z., Yin, S., Peng, H. (2024). Flexible phase change materials based on hexagonal boron nitride (hBN) surface modification and styrene-butadiene-styrene (SBS)/low-density polyethylene (LDPE) crosslinking for battery thermal management applications. Chemical Engineering Journal, 485, 150110. https://doi.org/10.1016/j.cej.2024.150110
- Patil, J. R., Mahanwar, P. A., Sundaramoorthy, E., Mundhe, G. S. (2023). A review of the thermal storage of phase change material, morphology, synthesis methods, characterization, and applications of microencapsulated phase change material. Journal of Polymer Engineering, 43 (4), 354–375. https://doi.org/10.1515/polyeng-2022-0254
- Wang, Z., Zhang, X., Xu, Y., Chen, G., Lin, F., Ding, H. (2021). Preparation and thermal properties of shape-stabilized composite phase change materials based on paraffin wax and carbon foam. Polymer, 237, 124361. https://doi.org/10.1016/j.polymer.2021.124361
- Firman, L. O. M., Rahmalina, D., Ismail, Rahman, R. A. (2023). Hybrid energy-temperature method (HETM): A low-cost apparatus and reliable method for estimating the thermal capacity of solid–liquid phase change material for heat storage system. HardwareX, 16, e00496. https://doi.org/10.1016/j.ohx.2023.e00496
- Suyitno, B. M., Anggrainy, R., Plamonia, N., Rahman, R. A. (2023). Preliminary characterization and thermal evaluation of a direct contact cascaded immiscible inorganic salt/high-density polyethylene as moderate temperature heat storage material. Results in Materials, 19, 100443. https://doi.org/10.1016/j.rinma.2023.100443
- Janghel, D., Karagadde, S., Saha, S. K. (2023). Measurement of shrinkage void and identification of solid-liquid phases in phase change materials: Ultrasound-based approach and simulated predictions. Applied Thermal Engineering, 223, 120048. https://doi.org/10.1016/j.applthermaleng.2023.120048
- Wu, M. Q., Wu, S., Cai, Y. F., Wang, R. Z., Li, T. X. (2021). Form-stable phase change composites: Preparation, performance, and applications for thermal energy conversion, storage and management. Energy Storage Materials, 42, 380–417. https://doi.org/10.1016/j.ensm.2021.07.019
- Cui, W., Li, X., Li, X., Lu, L., Ma, T., Wang, Q. (2022). Combined effects of nanoparticles and ultrasonic field on thermal energy storage performance of phase change materials with metal foam. Applied Energy, 309, 118465. https://doi.org/10.1016/j.apenergy.2021.118465
- Bastida, H., De la Cruz-Loredo, I., Ugalde-Loo, C. E. (2023). Effective estimation of the state-of-charge of latent heat thermal energy storage for heating and cooling systems using non-linear state observers. Applied Energy, 331, 120448. https://doi.org/10.1016/j.apenergy.2022.120448
- Rahmalina, D., Adhitya, D. C., Rahman, R. A., Ismail, I. (2021). Improvement the performance of composite PCM paraffin-based incorporate with volcanic ash as heat storage for low-temperature application. EUREKA: Physics and Engineering, 1, 53–61. https://doi.org/10.21303/2461-4262.2022.002055
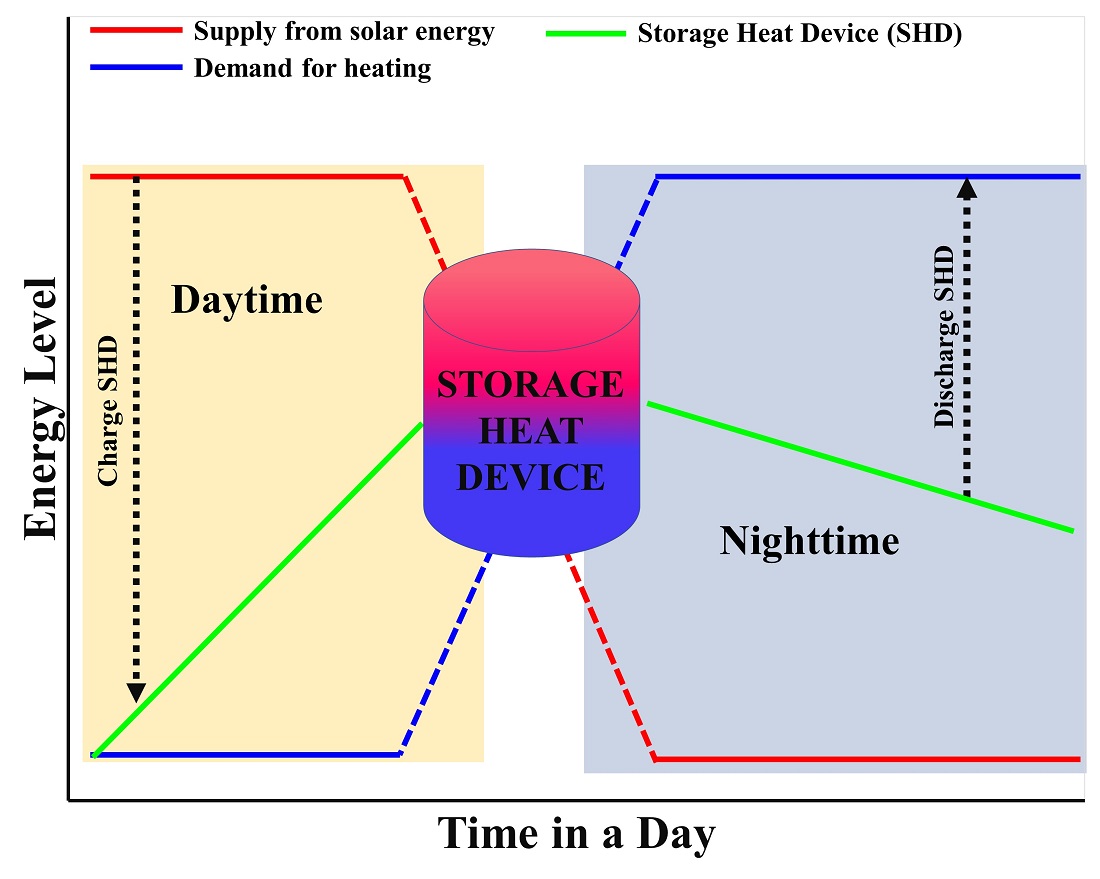
Downloads
Published
How to Cite
Issue
Section
License
Copyright (c) 2024 Reza Abdu Rahman, Sulistyo, Mohamad Said Kartono Tony Suryo Utomo, Robertus Dhimas Dhewangga Putra

This work is licensed under a Creative Commons Attribution 4.0 International License.
The consolidation and conditions for the transfer of copyright (identification of authorship) is carried out in the License Agreement. In particular, the authors reserve the right to the authorship of their manuscript and transfer the first publication of this work to the journal under the terms of the Creative Commons CC BY license. At the same time, they have the right to conclude on their own additional agreements concerning the non-exclusive distribution of the work in the form in which it was published by this journal, but provided that the link to the first publication of the article in this journal is preserved.
A license agreement is a document in which the author warrants that he/she owns all copyright for the work (manuscript, article, etc.).
The authors, signing the License Agreement with TECHNOLOGY CENTER PC, have all rights to the further use of their work, provided that they link to our edition in which the work was published.
According to the terms of the License Agreement, the Publisher TECHNOLOGY CENTER PC does not take away your copyrights and receives permission from the authors to use and dissemination of the publication through the world's scientific resources (own electronic resources, scientometric databases, repositories, libraries, etc.).
In the absence of a signed License Agreement or in the absence of this agreement of identifiers allowing to identify the identity of the author, the editors have no right to work with the manuscript.
It is important to remember that there is another type of agreement between authors and publishers – when copyright is transferred from the authors to the publisher. In this case, the authors lose ownership of their work and may not use it in any way.