Design of the predictive management and control system for combined propulsion complex
DOI:
https://doi.org/10.15587/1729-4061.2024.313627Keywords:
propulsion system, predictive control, distributed system, high-level controllerAbstract
The object of this researching is the process of maneuvering a sea-based vehicle under compressed conditions, which requires one hundred percent reserve of thrusters (THRs) of various modifications and locations. The main problem is the provision of energy-efficient control over the ship's motion at low speed in the horizontal plane using a high-level predictive controller. The hierarchy of the motion control system (MCS) is usually divided into several levels with the help of a high-level motion controller and the THR motor control distribution algorithm. This allows for a modular software structure where a high-level controller (HLC) can be designed without using comprehensive information about the THR motors. However, for a certain reference of THR configurations, such a decoupling can lead to reduced control performance due to the limitations of HLC regarding the physical constraints of the vessel and the behavior of MCS.
The main results of the researching are methods to improve control performance using a nonlinear model predictive control (MPC) as a basis for the designed motion controllers due to its optimized solution and ability to consider constraints. A decoupled system was implemented for two simple motor tasks showing dissociation problems. The shortcomings were eliminated through the development of a nonlinear MPC controller, which combines the motion controller and the distribution of control over THR motors. To preserve the discrete modularity of the control system and achieve adequate performance, a nonlinear MPC controller with time-varying constraints was designed. This has made it possible to take into account the current limitations of the THR control system, increase the accuracy of control, and reduce the response time of the system by 10 %.
References
- Budashko, V. (2017). Formalization of design for physical model of the azimuth thruster with two degrees of freedom by computational fluid dynamics methods. Eastern-European Journal of Enterprise Technologies, 3 (7 (87)), 40–49. https://doi.org/10.15587/1729-4061.2017.101298
- Fossen, T. I. (2021). Handbook of Marine Craft Hydrodynamics and Motion Control. Wiley. https://doi.org/10.1002/9781119575016
- van Goor, P., Hamel, T., Mahony, R. (2023). Constructive Equivariant Observer Design for Inertial Navigation. IFAC-PapersOnLine, 56 (2), 2494–2499. https://doi.org/10.1016/j.ifacol.2023.10.1229
- Maidana, R. G., Kristensen, S. D., Utne, I. B., Sørensen, A. J. (2023). Risk-based path planning for preventing collisions and groundings of maritime autonomous surface ships. Ocean Engineering, 290, 116417. https://doi.org/10.1016/j.oceaneng.2023.116417
- Bekker, J. R., Dou, S. X. (2002). A Packaged System Approach to DP Vessel Conversion. Dynamic Positioning Conference. Available at: http://dynamic-positioning.com/proceedings/dp2002/workboats_packaged_system.pdf
- Cozijn, H., Hallmann, R., Koop, A. (2010). Analysis of the velocities in the wake of an azimuthing thruster, using PIV measurements and CFD calculations. Dynamic positioning conference: thrusters session. Available at: https://dynamic-positioning.com/proceedings/dp2010/thrusters_cozijn.pdf
- Furmanik, M., Konvičný, D., Rafajdus, P. (2023). Low-Speed Sensorless Control for Six-Phase PMSM Based on Magnetic Anisotropy. Transportation Research Procedia, 74, 892–899. https://doi.org/10.1016/j.trpro.2023.11.222
- Hemalatha, N., Venkatesan, S., Kannan, R., Kannan, S., Bhuvanesh, A., Kamaraja, A. S. (2024). Sensorless speed and position control of permanent magnet BLDC motor using particle swarm optimization and ANFIS. Measurement: Sensors, 31, 100960. https://doi.org/10.1016/j.measen.2023.100960
- Sun, L. (2022). Low speed sensorless control method of brushless DC motor based on pulse high frequency voltage injection. Alexandria Engineering Journal, 61(8), 6457–6463. https://doi.org/10.1016/j.aej.2021.12.005
- Budashko, V., Sandler, A., Khniunin, S. (2023). Improving the method of linear-quadratic control over a physical model of vessel with azimuthal thrusters. Eastern-European Journal of Enterprise Technologies, 1 (2 (121)), 49–71. https://doi.org/10.15587/1729-4061.2023.273934
- de A. Fernandes, D., Sorensen, A. J., Donha, D. C. (2013). Trajectory Tracking Motion Control System for Observation Class ROVs. IFAC Proceedings Volumes, 46 (33), 251–256. https://doi.org/10.3182/20130918-4-jp-3022.00025
- Houska, B., Ferreau, H. J., Diehl, M. (2011). ACADO toolkit – An open‐source framework for automatic control and dynamic optimization. Optimal Control Applications and Methods, 32 (3), 298–312. https://doi.org/10.1002/oca.939
- Johansen, T. A., Fossen, T. I. (2013). Control allocation – A survey. Automatica, 49 (5), 1087–1103. https://doi.org/10.1016/j.automatica.2013.01.035
- Yari, E., Ghassemi, H. (2016). Hydrodynamic analysis of the surface-piercing propeller in unsteady open water condition using boundary element method. International Journal of Naval Architecture and Ocean Engineering, 8 (1), 22–37. https://doi.org/10.1016/j.ijnaoe.2015.09.002
- Budashko, V., Sandler, A., Shevchenko, V. (2022). Diagnosis of the Technical Condition of High-tech Complexes by Probabilistic Methods. TransNav, the International Journal on Marine Navigation and Safety of Sea Transportation, 16 (1), 105–111. https://doi.org/10.12716/1001.16.01.11
- Glad, T., Ljung, L. (2018). Control Theory. CRC Press. https://doi.org/10.1201/9781315274737
- Budashko, V. (2020). Thrusters Physical Model Formalization with regard to Situational and Identification Factors of Motion Modes. 2020 International Conference on Electrical, Communication, and Computer Engineering (ICECCE), 10, 1–6. https://doi.org/10.1109/icecce49384.2020.9179301
- Brezina, A., Thomas, S. (2013). Measurement of Static and Dynamic Performance Characteristics of Electric Propulsion Systems. 51st AIAA Aerospace Sciences Meeting Including the New Horizons Forum and Aerospace Exposition. https://doi.org/10.2514/6.2013-500
- Bucknall, R. W. G., Ciaramella, K. M. (2010). On the Conceptual Design and Performance of a Matrix Converter for Marine Electric Propulsion. IEEE Transactions on Power Electronics, 25 (6), 1497–1508. https://doi.org/10.1109/tpel.2009.2037961
- Zhong, Y., Yu, C., Bai, Y., Zeng, Z., Lian, L. (2024). Diving dynamics identification and motion prediction for marine crafts using field data. Journal of Ocean Engineering and Science, 9 (4), 391–400. https://doi.org/10.1016/j.joes.2023.12.001
- Abdessameud, A., Polushin, I. G., Tayebi, A. (2015). Motion coordination of thrust-propelled underactuated vehicles with intermittent and delayed communications. Systems & Control Letters, 79, 15–22. https://doi.org/10.1016/j.sysconle.2015.02.006
- Babadi, M. K., Ghassemi, H. (2013). Effect of hull form coefficients on the vessel sea-keeping performance. Journal of Marine Science and Technology. – 2013. – 11 p. https://doi.org/10.6119/JMST-013-0117-2
- Budashko, V., Sandler, A., Shevchenko, V. (2022). Optimization of the control system for an electric power system operating on a constant-power hyperbole. Eastern-European Journal of Enterprise Technologies, 1 (8 (115)), 6–17. https://doi.org/10.15587/1729-4061.2022.252172
- Carrera, A., Palomeras, N., Hurtós, N., Kormushev, P., Carreras, M. (2015). Cognitive system for autonomous underwater intervention. Pattern Recognition Letters, 67, 91–99. https://doi.org/10.1016/j.patrec.2015.06.010
- Budashko, V., Golikov, V. (2017). Theoretical-applied aspects of the composition of regression models for combined propulsion complexes based on data of experimental research. Eastern-European Journal of Enterprise Technologies, 4 (3 (88)), 11–20. https://doi.org/10.15587/1729-4061.2017.107244
- Myrhorod, V., Hvozdeva, I., Budashko, V. (2020). Multi-parameter Diagnostic Model of the Technical Conditions Changes of Ship Diesel Generator Sets. 2020 IEEE Problems of Automated Electrodrive. Theory and Practice (PAEP), 1895, 1–4. https://doi.org/10.1109/paep49887.2020.9240905
- Budashko, V., Shevchenko, V. (2021). The synthesis of control system to synchronize ship generator assemblies. Eastern-European Journal of Enterprise Technologies, 1 (2 (109)), 45–63. https://doi.org/10.15587/1729-4061.2021.225517
- Budashko, V., Shevchenko, V. (2021). Solving a task of coordinated control over a ship automated electric power system under a changing load. Eastern-European Journal of Enterprise Technologies, 2 (2 (110)), 54–70. https://doi.org/10.15587/1729-4061.2021.229033
- Sandler, A., Budashko, V. (2022). Improving tools for diagnosing technical condition of ship electric power installations. Eastern-European Journal of Enterprise Technologies, 5 (5 (119)), 25–33. https://doi.org/10.15587/1729-4061.2022.266267
- Sandler, A., Budashko, V., Khniunin, S., Bogach, V. (2023). Improving the mathematical model of a fiber-optic inclinometer for vibration diagnostics of elements in the propulsion system with sliding bearings. Eastern-European Journal of Enterprise Technologies, 5 (5 (125)), 24–31. https://doi.org/10.15587/1729-4061.2023.289773
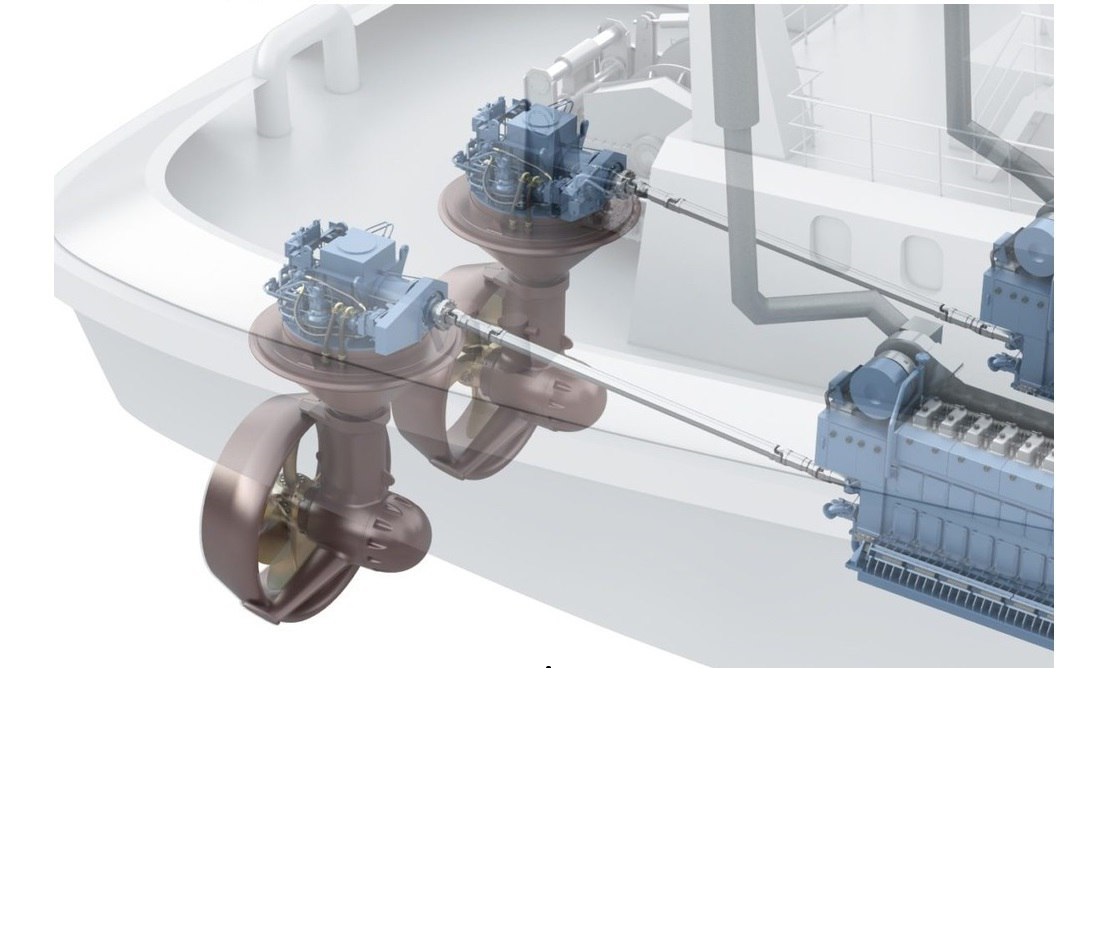
Downloads
Published
How to Cite
Issue
Section
License
Copyright (c) 2024 Vitalii Budashko, Albert Sandler, Sergii Khniunin, Valentyn Bogach

This work is licensed under a Creative Commons Attribution 4.0 International License.
The consolidation and conditions for the transfer of copyright (identification of authorship) is carried out in the License Agreement. In particular, the authors reserve the right to the authorship of their manuscript and transfer the first publication of this work to the journal under the terms of the Creative Commons CC BY license. At the same time, they have the right to conclude on their own additional agreements concerning the non-exclusive distribution of the work in the form in which it was published by this journal, but provided that the link to the first publication of the article in this journal is preserved.
A license agreement is a document in which the author warrants that he/she owns all copyright for the work (manuscript, article, etc.).
The authors, signing the License Agreement with TECHNOLOGY CENTER PC, have all rights to the further use of their work, provided that they link to our edition in which the work was published.
According to the terms of the License Agreement, the Publisher TECHNOLOGY CENTER PC does not take away your copyrights and receives permission from the authors to use and dissemination of the publication through the world's scientific resources (own electronic resources, scientometric databases, repositories, libraries, etc.).
In the absence of a signed License Agreement or in the absence of this agreement of identifiers allowing to identify the identity of the author, the editors have no right to work with the manuscript.
It is important to remember that there is another type of agreement between authors and publishers – when copyright is transferred from the authors to the publisher. In this case, the authors lose ownership of their work and may not use it in any way.