Assessment of assembly process complexity using material coefficients for handling and insertion
DOI:
https://doi.org/10.15587/1729-4061.2023.267430Keywords:
assembly, complexity index, material coefficient, material selection, handling, insertion, fasteningAbstract
Complexity principles are very important for reducing difficulty while at the same time continuously attaining the requirements of the product, process, and system. A crucial factor affecting the complexity of assembly is material selection. The material used for a product will be closely linked to the handling and insertion process. In a previous study, the methods used to select materials and assembly processes have been developed separately. In this study, those methods will be developed together into a single entity with respect to the complexity of each process. Scientific information about this matter has yet to be revealed, so it still requires intensive study. Hence, the study aims to promote a new way to measure the complexity of parts assembly by examining the material selection parameters. The proposed method involves material coefficients in establishing an assembly complexity index and consists of two phases. Numerical examples are provided to illustrate the suggested design comprehensively, which uses three material variants in piston products to calculate the complexity index of the assembly process. Variants with a small complexity index are ideal and facilitate the assembly process.
The study creates a material coefficient model to specify the assembly process, where each component has various coefficient values. The material coefficient describes the value of material characteristics related to the assembly method, namely the process of handling and insertion. The material selection requires a clear understanding of the assembly requirements for each component. The related material characteristics are density, fracture toughness, Young’s modulus, elastic limit, tensile strength, elongation, and hardness. Assessment of the complexity index using methods from previous studies obtained 6.02. Using the present method, 5.777 were obtained for variant 1 and 5.769 for the second variant. The mean complexity value is compatible with the material coefficient and assembly time
References
- Chow, W.-M. (2020). Assembly Line Design. CRC Press, 448. doi: https://doi.org/10.1201/9781003066477
- Laun, M., Czech, C., Hartmann, U., Terschüren, C., Harth, V., Karamanidis, K., Friemert, D. (2022). The acceptance of smart glasses used as side-by-side instructions for complex assembly tasks is highly dependent on the device model. International Journal of Industrial Ergonomics, 90, 103316. doi: https://doi.org/10.1016/j.ergon.2022.103316
- Panhalkar, N., Paul, R., Anand, S. (2014). Optimization of Automobile Assembly Process to Reduce Assembly Time. Computer-Aided Design and Applications, 11, S54–S60. doi: https://doi.org/10.1080/16864360.2014.914410
- Falck, A.-C., Örtengren, R., Rosenqvist, M., Söderberg, R. (2016). Proactive assessment of basic complexity in manual assembly: development of a tool to predict and control operator-induced quality errors. International Journal of Production Research, 55 (15), 4248–4260. doi: https://doi.org/10.1080/00207543.2016.1227103
- Sudhoff, M., Schüler, P., Herzog, M., Kuhlenkötter, B. (2022). Proving the Applicability of Assembly Complexity Measures for Process Time Prediction of Customer-specific Production. Procedia CIRP, 107, 381–386. doi: https://doi.org/10.1016/j.procir.2022.04.062
- Falck, A.-C., Tarrar, M., Mattsson, S., Andersson, L., Rosenqvist, M., Söderberg, R. (2017). Assessment of manual assembly complexity: a theoretical and empirical comparison of two methods. International Journal of Production Research, 55 (24), 7237–7250. doi: https://doi.org/10.1080/00207543.2017.1330571
- ElMaraghy, W., ElMaraghy, H., Tomiyama, T., Monostori, L. (2012). Complexity in engineering design and manufacturing. CIRP Annals, 61 (2), 793–814. doi: https://doi.org/10.1016/j.cirp.2012.05.001
- Wang, K., Xie, G., Xiang, J., Li, T., Peng, Y., Wang, J., Zhang, H. (2022). Materials selection of 3D printed polyamide-based composites at different strain rates: A case study of automobile front bumpers. Journal of Manufacturing Processes, 84, 1449–1462. doi: https://doi.org/10.1016/j.jmapro.2022.11.024
- Dammann, M., Schüppstuhl, T. (2018). Automated selection and assembly of sets of blades for jet engine compressors and turbines. Procedia Manufacturing, 16, 53–60. doi: https://doi.org/10.1016/j.promfg.2018.10.159
- Petunina, I., Zrazhevskiy, A., Kuzmin, O. (2022). Manufacturing Technology of complex non-assembly mechanisms with movable parts in Civil Engineering. CIRP Journal of Manufacturing Science and Technology, 37, 227–232. doi: https://doi.org/10.1016/j.cirpj.2022.01.016
- Zhang, J., Wang, S., He, W., Li, J., Wu, S., Huang, J. et al. (2022). Augmented reality material management system based on post-processing of aero-engine blade code recognition. Journal of Manufacturing Systems, 65, 564–578. doi: https://doi.org/10.1016/j.jmsy.2022.10.006
- Ukala, A. N., Sunmola, F. T. (2020). A Rule-Based Approach for Product Assembly Complexity Review in the Context of Virtual Engineering. Procedia Manufacturing, 51, 557–564. doi: https://doi.org/10.1016/j.promfg.2020.10.078
- Facchini, F., Cavallo, D., Mummolo, G. (2022). A Model to Estimate Operators’ Performance in Accomplishing Assembly Tasks. Industrial Engineering and Operations Management, 193–205. doi: https://doi.org/10.1007/978-3-031-14763-0_16
- Li-li, L., Kun, C., Jian-min, G., Jun-kong, L., Zhi-yong, G., Hong-wei, D. (2022). Research on optimizing-assembly and optimizing-adjustment technologies of aero-engine fan rotor blades. Advanced Engineering Informatics, 51, 101506. doi: https://doi.org/10.1016/j.aei.2021.101506
- Vučetić, N., Jovičić, G., Krstić, B., Živković, M., Milovanović, V., Kačmarčik, J., Antunović, R. (2020). Research of an aircraft engine cylinder assembly integrity assessment – Thermomechanical FEM analysis. Engineering Failure Analysis, 111, 104453. doi: https://doi.org/10.1016/j.engfailanal.2020.104453
- Wang, H., Gu, T., Jin, M., Zhao, R., Wang, G. (2018). The complexity measurement and evolution analysis of supply chain network under disruption risks. Chaos, Solitons & Fractals, 116, 72–78. doi: https://doi.org/10.1016/j.chaos.2018.09.018
- Grogan, P. T. (2021). Perception of complexity in engineering design. Systems Engineering, 24 (4), 221–233. doi: https://doi.org/10.1002/sys.21574
- Alkan, B., Vera, D. A., Ahmad, M., Ahmad, B., Harrison, R. (2018). Complexity in manufacturing systems and its measures: a literature review. European J. of Industrial Engineering, 12 (1), 116. doi: https://doi.org/10.1504/ejie.2018.089883
- Domoto, Y., Fujita, M. (2022). Self-assembly of nanostructures with high complexity based on metal⋯unsaturated-bond coordination. Coordination Chemistry Reviews, 466, 214605. doi: https://doi.org/10.1016/j.ccr.2022.214605
- Mallick, P. K. (Ed.) (2021). Materials, Design and Manufacturing for Lightweight Vehicles. Elsevier. doi: https://doi.org/10.1016/C2018-0-04153-5
- Loor, R. B. S., Gómez, J. M., Hoyos, J. C. R., Cedeño, E. A. Ll. (2020). Selection of materials by multi-criteria methods applied to the side of a self-supporting structure for light vehicles. International Journal of Mathematics in Operational Research, 16 (2), 139. doi: https://doi.org/10.1504/ijmor.2020.105844
- Ghavami, S. M. (2019). Multi-criteria spatial decision support system for identifying strategic roads in disaster situations. International Journal of Critical Infrastructure Protection, 24, 23–36. doi: https://doi.org/10.1016/j.ijcip.2018.10.004
- Emovon, I., Oghenenyerovwho, O. S. (2020). Application of MCDM method in material selection for optimal design: A review. Results in Materials, 7, 100115. doi: https://doi.org/10.1016/j.rinma.2020.100115
- Rahim, A. A., Musa, S. N., Ramesh, S., Lim, M. K. (2020). A systematic review on material selection methods. Proceedings of the Institution of Mechanical Engineers, Part L: Journal of Materials: Design and Applications, 234 (7), 1032–1059. doi: https://doi.org/10.1177/1464420720916765
- Athawale, V. M., Chakraborty, S. (2012). Material selection using multi-criteria decision-making methods: a comparative study. Proceedings of the Institution of Mechanical Engineers, Part L: Journal of Materials: Design and Applications, 226 (4), 266–285. doi: https://doi.org/10.1177/1464420712448979
- Ashby, M. F., Shercliff, H., Cebon, D. (2019). Materials: Engineering, Science, Processing and Design. Elsevier Butterworth-Heinemann, 806.
- Rahim, A. A., Musa, S. N., Ramesh, S., Lim, M. K. (2021). Development of a fuzzy-TOPSIS multi-criteria decision-making model for material selection with the integration of safety, health and environment risk assessment. Proceedings of the Institution of Mechanical Engineers, Part L: Journal of Materials: Design and Applications, 235 (7), 1532–1550. doi: https://doi.org/10.1177/1464420721994269
- Maleque, M. A., Salit, M. S. (2013). Materials Selection and Design. Springer, 120. doi: https://doi.org/10.1007/978-981-4560-38-2
- Tian, G., Zhang, H., Feng, Y., Wang, D., Peng, Y., Jia, H. (2018). Green decoration materials selection under interior environment characteristics: A grey-correlation based hybrid MCDM method. Renewable and Sustainable Energy Reviews, 81, 682–692. doi: https://doi.org/10.1016/j.rser.2017.08.050
- Chatterjee, P., Chakraborty, S. (2012). Material selection using preferential ranking methods. Materials & Design, 35, 384–393. doi: https://doi.org/10.1016/j.matdes.2011.09.027
- Samy, S. N., ElMaraghy, H. A. (2012). Complexity mapping of the product and assembly system. Assembly Automation, 32 (2), 135–151. doi: https://doi.org/10.1108/01445151211212299
- Ashby, M. F. (2016). Materials Selection in Mechanical Design. Elsevier Butterworth-Heinemann.
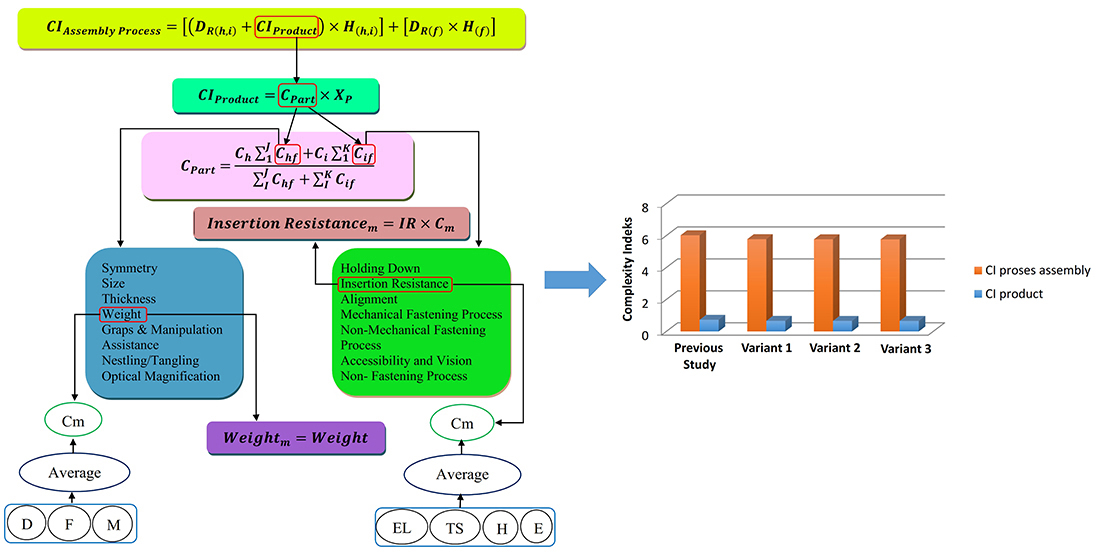
Downloads
Published
How to Cite
Issue
Section
License
Copyright (c) 2023 Nelce Dominggas Muskita, Rudy Soenoko, Achmad As’ad Sonief, Moch. Agus Choiron

This work is licensed under a Creative Commons Attribution 4.0 International License.
The consolidation and conditions for the transfer of copyright (identification of authorship) is carried out in the License Agreement. In particular, the authors reserve the right to the authorship of their manuscript and transfer the first publication of this work to the journal under the terms of the Creative Commons CC BY license. At the same time, they have the right to conclude on their own additional agreements concerning the non-exclusive distribution of the work in the form in which it was published by this journal, but provided that the link to the first publication of the article in this journal is preserved.
A license agreement is a document in which the author warrants that he/she owns all copyright for the work (manuscript, article, etc.).
The authors, signing the License Agreement with TECHNOLOGY CENTER PC, have all rights to the further use of their work, provided that they link to our edition in which the work was published.
According to the terms of the License Agreement, the Publisher TECHNOLOGY CENTER PC does not take away your copyrights and receives permission from the authors to use and dissemination of the publication through the world's scientific resources (own electronic resources, scientometric databases, repositories, libraries, etc.).
In the absence of a signed License Agreement or in the absence of this agreement of identifiers allowing to identify the identity of the author, the editors have no right to work with the manuscript.
It is important to remember that there is another type of agreement between authors and publishers – when copyright is transferred from the authors to the publisher. In this case, the authors lose ownership of their work and may not use it in any way.