Condensation heat removal due to the combined impact of natural convection and radiative cooling
DOI:
https://doi.org/10.15587/1729-4061.2023.273607Keywords:
radiation cooling, refrigerating machine, condenser, natural air circulation, energy efficiencyAbstract
The work examines the heat exchange characteristics of a condenser in which heat removal of the refrigerant condensation occurs due to natural convection and radiation cooling. The heat exchanger is designed to reduce energy costs for the removal of condensation heat. Unlike traditional air cooling condensers, it uses radiation cooling, a method of heat removal based on its transmission in the form of infrared radiation through the planet’s atmosphere into the surrounding outer space. A method for calculating the thickness of the radiating plate has been developed. To minimize material consumption and cost, the distance between the tubes is reduced to 40 mm, and the thickness of the aluminum radiating plate is reduced to 0.32 mm. The inner diameter of the coolant channels is 1 mm.
For the experimental study of the condenser, an experimental stand working on R134a refrigerant was developed. Theoretical and experimental studies of heat transfer have been carried out. The heat transfer coefficient of the heat exchanger, reduced to the area of the radiating surface, was from 10.3±1.36 to 18.7±2.47 W·m–2·°C–1, when the condensation temperature was 12.8...21.9 °С higher than the temperature of atmospheric air. The operability of the condenser is shown both during the day and at night, in the presence of precipitation in the form of rain and snow, and a high level of cloudiness.
The material capacity and filling of the refrigerant in the condenser are comparable to the characteristics of air-cooled condensers with forced air circulation. At the same time, it does not consume electricity. It can be used in stationary refrigeration systems (in data processing centers, commercial refrigeration equipment, air conditioners) to increase their energy efficiency
References
- Dobson, R. (2005). Thermal modelling of a night sky radiation cooling system. Journal of Energy in Southern Africa, 16 (2), 20–31. doi: https://doi.org/10.17159/2413-3051/2005/v16i2a3184
- Meir, M. G., Rekstad, J. B., LØvvik, O. M. (2002). A study of a polymer-based radiative cooling system. Solar Energy, 73 (6), 403–417. doi: https://doi.org/10.1016/s0038-092x(03)00019-7
- Anderson, T., Duke, M., Carson, J. (2013). Performance of an unglazed solar collector for radiant cooling. Australian Solar Cooling 2013 Conference. Available at: https://openrepository.aut.ac.nz/handle/10292/5651?show=full
- Vidhi, R. (2018). A Review of Underground Soil and Night Sky as Passive Heat Sink: Design Configurations and Models. Energies, 11 (11), 2941. doi: https://doi.org/10.3390/en11112941
- Bagiorgas, H. S., Mihalakakou, G. (2008). Experimental and theoretical investigation of a nocturnal radiator for space cooling. Renewable Energy, 33 (6), 1220–1227. doi: https://doi.org/10.1016/j.renene.2007.04.015
- Hollick, J. (2012). Nocturnal Radiation Cooling Tests. Energy Procedia, 30, 930–936. doi: https://doi.org/10.1016/j.egypro.2012.11.105
- Tsoy, A. P., Baranenko, A. V., Granovsky, A. S., Tsoy, D. A., Dzhamasheva, R. A. (2020). Energy efficiency analysis of a combined cooling system with night radiative cooling. AIP Conference Proceedings. doi: https://doi.org/10.1063/5.0026908
- Karagusov, V. I., Serdyuk, V. S., Kolpakov, I. S., Nemykin, V. A., Pogulyaev, I. N. (2018). Experimental determination of rate and direction of heat flow of the radiation life – Support system with vacuum heat insulation. AIP Conference Proceedings. doi: https://doi.org/10.1063/1.5051876
- Goldstein, E. A., Raman, A. P., Fan, S. (2017). Sub-ambient non-evaporative fluid cooling with the sky. Nature Energy, 2 (9). doi: https://doi.org/10.1038/nenergy.2017.143
- Goldstein, E. A., Nasuta, D., Li, S., Martin, C., Raman, A. (2018). Free Subcooling with the Sky: Improving the efficiency of air conditioning systems. 17th International Refrigeration and Air Conditioning Conference at Purdue. Available at: https://docs.lib.purdue.edu/iracc/1913/
- Tsoy, A., Granovskiy, A., Tsoy, D., Koretskiy, D. (2022). Cooling capacity of experimental system with natural refrigerant circulation and condenser radiative cooling. Eastern-European Journal of Enterprise Technologies, 2 (8 (116)), 45–53. doi: https://doi.org/10.15587/1729-4061.2022.253651
- Ezekwe, C. I. (1990). Performance of a heat pipe assisted night sky radiative cooler. Energy Conversion and Management, 30 (4), 403–408. doi: https://doi.org/10.1016/0196-8904(90)90041-v
- Yu, C., Shen, D., Jiang, Q., He, W., Yu, H., Hu, Z. et al. (2019). Numerical and Experimental Study on the Heat Dissipation Performance of a Novel System. Energies, 13 (1), 106. doi: https://doi.org/10.3390/en13010106
- Kim, M.-H., Lee, S. Y., Mehendale, S. S., Webb, R. L. (2003). Microchannel Heat Exchanger Design for Evaporator and Condenser Applications. Advances in Heat Transfer, 297–429. doi: https://doi.org/10.1016/s0065-2717(03)37004-2
- Butrymowicz, D., Śmierciew, K., Gagan, J., Dudar, A., Łukaszuk, M., Zou, H., Łapiński, A. (2022). Investigations of Performance of Mini-Channel Condensers and Evaporators for Propane. Sustainability, 14 (21), 14249. doi: https://doi.org/10.3390/su142114249
- Boeng, J., Rametta, R. S., Melo, C., Hermes, C. J. L. (2020). Thermal-hydraulic characterization and system-level optimization of microchannel condensers for household refrigeration applications. Thermal Science and Engineering Progress, 20, 100479. doi: https://doi.org/10.1016/j.tsep.2020.100479
- Walton, G. (1983). Thermal Analysis Research Program. Washington, 292. Available at: https://ntrl.ntis.gov/NTRL/dashboard/searchResults/titleDetail/PB83194225.xhtml
- Samuel, D. G. L., Nagendra, S. M. S., Maiya, M. P. (2013). Passive alternatives to mechanical air conditioning of building: A review. Building and Environment, 66, 54–64. doi: https://doi.org/10.1016/j.buildenv.2013.04.016
- Zhao, D., Aili, A., Zhai, Y., Xu, S., Tan, G., Yin, X., Yang, R. (2019). Radiative sky cooling: Fundamental principles, materials, and applications. Applied Physics Reviews, 6 (2), 021306. doi: https://doi.org/10.1063/1.5087281
- Zhao, D., Aili, A., Zhai, Y., Lu, J., Kidd, D., Tan, G. et al. (2019). Subambient Cooling of Water: Toward Real-World Applications of Daytime Radiative Cooling. Joule, 3 (1), 111–123. doi: https://doi.org/10.1016/j.joule.2018.10.006
- Mukhachev, G. A., Schukin, V. K. (1991). Termodinamika i teploperedacha. Moscow: Vysshaya shkola.
- Bohdal, T., Charun, H., Sikora, M. (2012). Heat transfer during condensation of refrigerants in tubular minichannels. Archives of Thermodynamics, 33 (2), 3–22. doi: https://doi.org/10.2478/v10173-012-0008-x
- Isachenko, V. P., Osipova, V. A., Sukomel, A. (1975). Teploperedacha. Moscow: Energiya, 315.
- Tevar, J. A. F., Castaño, S., Marijuán, A. G., Heras, M. R., Pistono, J. (2015). Modelling and experimental analysis of three radioconvective panels for night cooling. Energy and Buildings, 107, 37–48. doi: https://doi.org/10.1016/j.enbuild.2015.07.027
- Bell, I. H., Wronski, J., Quoilin, S., Lemort, V. (2014). Pure and Pseudo-pure Fluid Thermophysical Property Evaluation and the Open-Source Thermophysical Property Library CoolProp. Industrial & Engineering Chemistry Research, 53 (6), 2498–2508. doi: https://doi.org/10.1021/ie4033999
- Orzechowski, T., Stokowiec, K. (2014). Free convection on a refrigerator’s condenser. EPJ Web of Conferences, 67, 02089. doi: https://doi.org/10.1051/epjconf/20146702089
- Saleh, A. A. M. (2019). Correlation of overall heat transfer coefficient in the three zones of wire and tube condenser. Journal of Mechanical Engineering Research and Developments, 42 (1), 96–103. doi: https://doi.org/10.26480/jmerd.01.2019.96.103
- Ahmad, M. I., Jarimi, H., Riffat, S. (2019). Nocturnal Cooling Technology for Building Applications. Springer, 70. https://doi.org/10.1007/978-981-13-5835-7
- Aili, A., Zhao, D., Lu, J., Zhai, Y., Yin, X., Tan, G., Yang, R. (2019). A kW-scale, 24-hour continuously operational, radiative sky cooling system: Experimental demonstration and predictive modeling. Energy Conversion and Management, 186, 586–596. doi: https://doi.org/10.1016/j.enconman.2019.03.006
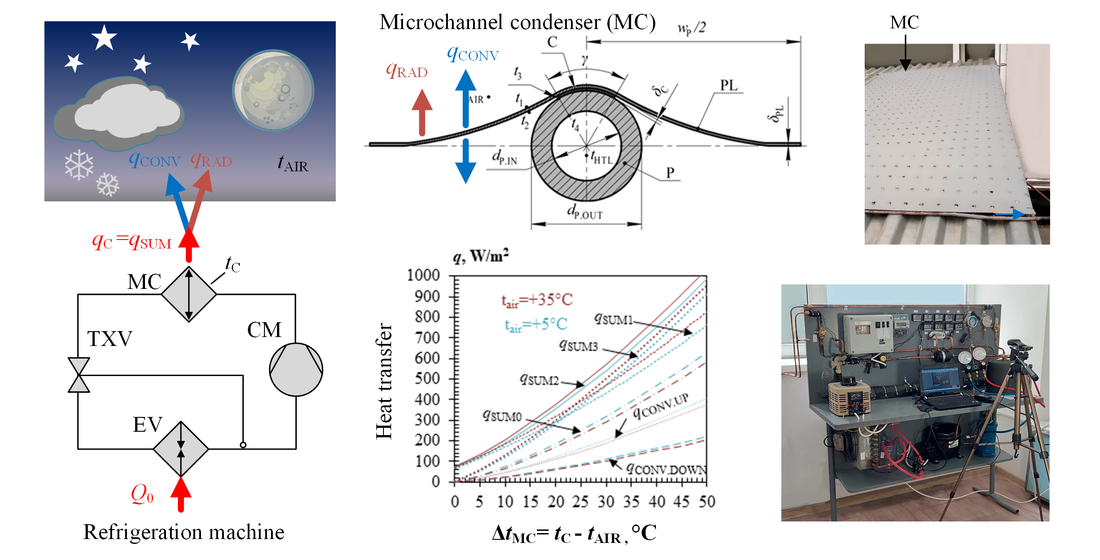
Downloads
Published
How to Cite
Issue
Section
License
Copyright (c) 2023 Alexandr Tsoy, Alexandr Granovskiy, Baurzhan Nurakhmetov, Dmitriy Koretskiy, Diana Tsoy-Davis, Nikita Veselskiy

This work is licensed under a Creative Commons Attribution 4.0 International License.
The consolidation and conditions for the transfer of copyright (identification of authorship) is carried out in the License Agreement. In particular, the authors reserve the right to the authorship of their manuscript and transfer the first publication of this work to the journal under the terms of the Creative Commons CC BY license. At the same time, they have the right to conclude on their own additional agreements concerning the non-exclusive distribution of the work in the form in which it was published by this journal, but provided that the link to the first publication of the article in this journal is preserved.
A license agreement is a document in which the author warrants that he/she owns all copyright for the work (manuscript, article, etc.).
The authors, signing the License Agreement with TECHNOLOGY CENTER PC, have all rights to the further use of their work, provided that they link to our edition in which the work was published.
According to the terms of the License Agreement, the Publisher TECHNOLOGY CENTER PC does not take away your copyrights and receives permission from the authors to use and dissemination of the publication through the world's scientific resources (own electronic resources, scientometric databases, repositories, libraries, etc.).
In the absence of a signed License Agreement or in the absence of this agreement of identifiers allowing to identify the identity of the author, the editors have no right to work with the manuscript.
It is important to remember that there is another type of agreement between authors and publishers – when copyright is transferred from the authors to the publisher. In this case, the authors lose ownership of their work and may not use it in any way.