Design of ammonia sensor based on ZnO for analyzing hazards at critical infrastructure
DOI:
https://doi.org/10.15587/1729-4061.2024.298512Keywords:
ZnO, gas sensor, magnetron sputtering, standard temperature, ammoniaAbstract
A gas sensor based on ZnO has been designed, which demonstrates sensitivity to NH3 under standard conditions (temperature, 25 °С; pressure, 101.3 kPa). The experimental sample was manufactured by magnetron sputtering at direct current. A VUP-5M vacuum unit with an original material-saving magnetron was used to produce ZnO films. To analyze the efficiency of the gas sensor to ammonia (NH3) under standard conditions, its operating characteristics were studied. The concentration of NH3 for investigating operating characteristics was chosen at the level of 25 ppm. To determine the resistivity of the contacts of the instrument structure, the current-voltage characteristics of the gas sensor were examined in the voltage range between −100 and +100 V. Based on the results of investigating the current-voltage characteristics, which have a linear character, the resistivity of the contacts was confirmed. To study the sensitivity of the gas sensor to the target gas, the change in resistance of the sensitive layer of the gas sensor under the influence of NH3 with a concentration of 25 ppm under standard conditions was explored. The study results demonstrated the high sensitivity of the gas sensor to the target gas – at the level of 229 relative units. The investigation of the response and recovery time of the gas sensor showed that the ZnO-based gas sensor has a response and recovery time of 20 and 26 s, respectively. The selectivity of the ZnO-based gas sensor was studied. The selectivity study was carried out by determining the sensitivity of the gas sensor in the presence of vapors of various gases, namely methanol, ethanol, acetone. The study results showed that the reaction to ammonia is selective compared to the reaction to other gases. The results of examining the working characteristics of the ammonia gas sensor demonstrate the high efficiency of its application under standard conditions and a low concentration of the target gas
References
- Sadkovyi, V., Pospelov, B., Andronov, V., Rybka, E., Krainiukov, O., Rud, A. et al. (2020). Construction of a method for detecting arbitrary hazard pollutants in the atmospheric air based on the structural function of the current pollutant concentrations. Eastern-European Journal of Enterprise Technologies, 6 (10 (108)), 14–22. https://doi.org/10.15587/1729-4061.2020.218714
- Kwak, D., Lei, Y., Maric, R. (2019). Ammonia gas sensors: A comprehensive review. Talanta, 204, 713–730. https://doi.org/10.1016/j.talanta.2019.06.034
- Arnone, M., Koppisch, D., Smola, T., Gabriel, S., Verbist, K., Visser, R. (2015). Hazard banding in compliance with the new Globally Harmonised System (GHS) for use in control banding tools. Regulatory Toxicology and Pharmacology, 73 (1), 287–295. https://doi.org/10.1016/j.yrtph.2015.07.014
- Corkery, G., Ward, S., Kenny, C., Hemmingway, P. (2013). Monitoring environmental parameters in poultry production facilities. Computer Aided Process Engineering, CAPE Forum 2013. Graz University of Technology.
- Popov, O., Ivaschenko, T., Markina, L., Yatsyshyn, T., Iatsyshyn, A., Lytvynenko, O. (2023). Peculiarities of Specialized Software Tools Used for Consequences Assessment of Accidents at Chemically Hazardous Facilities. Systems, Decision and Control in Energy V, 779–798. https://doi.org/10.1007/978-3-031-35088-7_45
- Pospelov, B., Rybka, E., Meleshchenko, R., Krainiukov, O., Biryukov, I., Butenko, T. et al. (2021). Short-term fire forecast based on air state gain recurrence and zero-order brown model. Eastern-European Journal of Enterprise Technologies, 3 (10 (111)), 27–33. https://doi.org/10.15587/1729-4061.2021.233606
- Vambol, S., Vambol, V., Kondratenko, O., Suchikova, Y., Hurenko, O. (2017). Assessment of improvement of ecological safety of power plants by arranging the system of pollutant neutralization. Eastern-European Journal of Enterprise Technologies, 3 (10 (87)), 63–73. https://doi.org/10.15587/1729-4061.2017.102314
- Minska, N., Hvozd, V., Shevchenko, O., Slepuzhnikov, Y., Murasov, R., Khrystych, V. et al. (2023). Devising technological solutions for gas sensors based on zinc oxide for use at critical infrastructure facilities. Eastern-European Journal of Enterprise Technologies, 4 (5 (124)), 34–40. https://doi.org/10.15587/1729-4061.2023.286546
- Miasoiedova, A., Minska, N., Shevchenko, R., Azarenkо, O., Lukashenko, V., Kyrychenko, O. et al. (2023). Improving the manufacturing technology of sensing gas sensors based on zinc oxide by using the method of magnetron sputtering on direct current. Eastern-European Journal of Enterprise Technologies, 2 (5 (122)), 31–37. https://doi.org/10.15587/1729-4061.2023.277428
- Vambol, S., Bogdanov, I., Vambol, V., Suchikova, Y., Kondratenko, O., Hurenko, O., Onishchenko, S. (2017). Research into regularities of pore formation on the surface of semiconductors. Eastern-European Journal of Enterprise Technologies, 3 (5 (87)), 37–44. https://doi.org/10.15587/1729-4061.2017.104039
- Peeters, R., Berden, G., Apituley, A., Meijer, G. (2000). Open-path trace gas detection of ammonia based on cavity-enhanced absorption spectroscopy. Applied Physics B, 71 (2), 231–236. https://doi.org/10.1007/s003400000302
- Liu, X., Cheng, S., Liu, H., Hu, S., Zhang, D., Ning, H. (2012). A Survey on Gas Sensing Technology. Sensors, 12 (7), 9635–9665. https://doi.org/10.3390/s120709635
- Xiong, L., Compton, R. G. (2014). Amperometric Gas detection: A Review. International Journal of Electrochemical Science, 9 (12), 7152–7181. https://doi.org/10.1016/s1452-3981(23)10957-6
- Huang, J., Wang, J., Gu, C., Yu, K., Meng, F., Liu, J. (2009). A novel highly sensitive gas ionization sensor for ammonia detection. Sensors and Actuators A: Physical, 150 (2), 218–223. https://doi.org/10.1016/j.sna.2009.01.008
- Zhao, Y. M., Zhu, Y. Q. (2009). Room temperature ammonia sensing properties of W18O49 nanowires. Sensors and Actuators B: Chemical, 137 (1), 27–31. https://doi.org/10.1016/j.snb.2009.01.004
- Wang, G., Ji, Y., Huang, X., Yang, X., Gouma, P.-I., Dudley, M. (2006). Fabrication and Characterization of Polycrystalline WO3 Nanofibers and Their Application for Ammonia Sensing. The Journal of Physical Chemistry B, 110 (47), 23777–23782. https://doi.org/10.1021/jp0635819
- Prasad, A. K., Gouma, P. I., Kubinski, D. J., Visser, J. H., Soltis, R. E., Schmitz, P. J. (2003). Reactively sputtered MoO3 films for ammonia sensing. Thin Solid Films, 436 (1), 46–51. https://doi.org/10.1016/s0040-6090(03)00524-8
- Tu, Y., Kyle, C., Luo, H., Zhang, D.-W., Das, A., Briscoe, J. et al. (2020). Ammonia Gas Sensor Response of a Vertical Zinc Oxide Nanorod-Gold Junction Diode at Room Temperature. ACS Sensors, 5 (11), 3568–3575. https://doi.org/10.1021/acssensors.0c01769
- Seekaew, Y., Pon-On, W., Wongchoosuk, C. (2019). Ultrahigh Selective Room-Temperature Ammonia Gas Sensor Based on Tin–Titanium Dioxide/reduced Graphene/Carbon Nanotube Nanocomposites by the Solvothermal Method. ACS Omega, 4 (16), 16916–16924. https://doi.org/10.1021/acsomega.9b02185
- Maity, A., Raychaudhuri, A. K., Ghosh, B. (2019). High sensitivity NH3 gas sensor with electrical readout made on paper with perovskite halide as sensor material. Scientific Reports, 9 (1). https://doi.org/10.1038/s41598-019-43961-6
- Husain, A. (2021). Electrical conductivity based ammonia, methanol and acetone vapour sensing studies on newly synthesized polythiophene/molybdenum oxide nanocomposite. Journal of Science: Advanced Materials and Devices, 6 (4), 528–537. https://doi.org/10.1016/j.jsamd.2021.07.002
- Seekaew, Y., Lokavee, S., Phokharatkul, D., Wisitsoraat, A., Kerdcharoen, T., Wongchoosuk, C. (2014). Low-cost and flexible printed graphene–PEDOT:PSS gas sensor for ammonia detection. Organic Electronics, 15 (11), 2971–2981. https://doi.org/10.1016/j.orgel.2014.08.044
- Kumar, L., Rawal, I., Kaur, A., Annapoorni, S. (2017). Flexible room temperature ammonia sensor based on polyaniline. Sensors and Actuators B: Chemical, 240, 408–416. https://doi.org/10.1016/j.snb.2016.08.173
- Zhao, S., Shen, Y., Yan, X., Zhou, P., Yin, Y., Lu, R. et al. (2019). Complex-surfactant-assisted hydrothermal synthesis of one-dimensional ZnO nanorods for high-performance ethanol gas sensor. Sensors and Actuators B: Chemical, 286, 501–511. https://doi.org/10.1016/j.snb.2019.01.127
- Xuan, J., Zhao, G., Sun, M., Jia, F., Wang, X., Zhou, T. et al. (2020). Low-temperature operating ZnO-based NO2 sensors: a review. RSC Advances, 10 (65), 39786–39807. https://doi.org/10.1039/d0ra07328h
- Danchenko, Y., Andronov, V., Barabash, E., Obigenko, T., Rybka, E., Meleshchenko, R., Romin, A. (2017). Research of the intramolecular interactions and structure in epoxyamine composites with dispersed oxides. Eastern-European Journal of Enterprise Technologies, 6 (12 (90)), 4–12. https://doi.org/10.15587/1729-4061.2017.118565
- Deyneko, N., Kovalev, P., Semkiv, O., Khmyrov, I., Shevchenko, R. (2019). Development of a technique for restoring the efficiency of film ITO/CdS/CdTe/Cu/Au SCs after degradation. Eastern-European Journal of Enterprise Technologies, 1 (5 (97)), 6–12. https://doi.org/10.15587/1729-4061.2019.156565
- Deyneko, N., Kryvulkin, I., Matiushenko, M., Tarasenko, O., Khmyrov, I., Khmyrova, A., Shevchenko, R. (2019). Investigation of photoelectric converters with a base cadmium telluride layer with a decrease in its thickness for tandem and two-sided sensitive instrument structures. EUREKA: Physics and Engineering, 5, 73–80. https://doi.org/10.21303/2461-4262.2019.001002
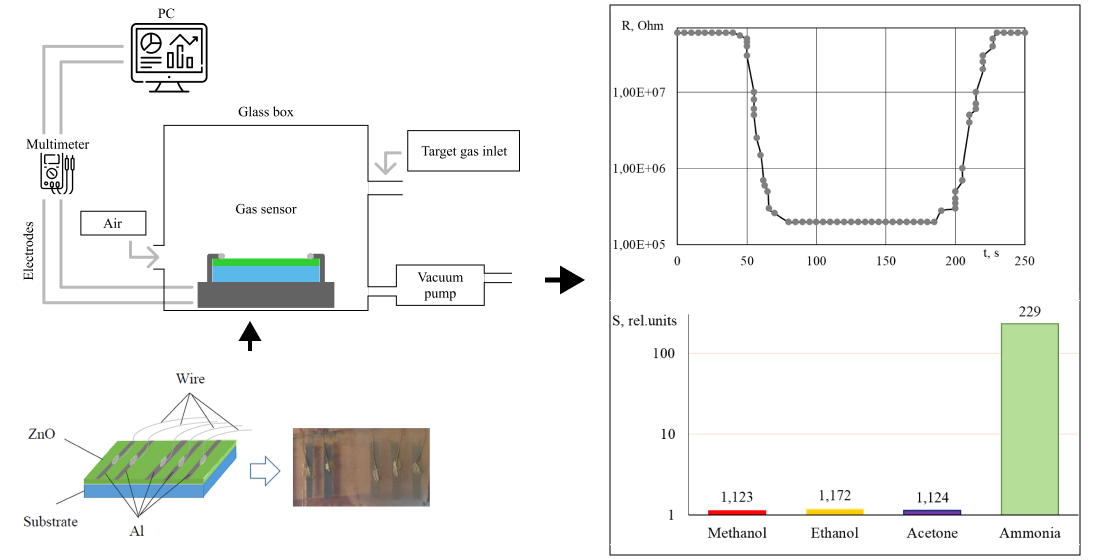
Downloads
Published
How to Cite
Issue
Section
License
Copyright (c) 2024 Natalia Minska, Oleh Bas, Viktor Hvozd, Oleksandr Hryhorenko, Alexander Levterov, Murat Maliarov, Mykola Matiushenko, Serhii Tarasov, Roman Chernysh, Olga Shevchenko

This work is licensed under a Creative Commons Attribution 4.0 International License.
The consolidation and conditions for the transfer of copyright (identification of authorship) is carried out in the License Agreement. In particular, the authors reserve the right to the authorship of their manuscript and transfer the first publication of this work to the journal under the terms of the Creative Commons CC BY license. At the same time, they have the right to conclude on their own additional agreements concerning the non-exclusive distribution of the work in the form in which it was published by this journal, but provided that the link to the first publication of the article in this journal is preserved.
A license agreement is a document in which the author warrants that he/she owns all copyright for the work (manuscript, article, etc.).
The authors, signing the License Agreement with TECHNOLOGY CENTER PC, have all rights to the further use of their work, provided that they link to our edition in which the work was published.
According to the terms of the License Agreement, the Publisher TECHNOLOGY CENTER PC does not take away your copyrights and receives permission from the authors to use and dissemination of the publication through the world's scientific resources (own electronic resources, scientometric databases, repositories, libraries, etc.).
In the absence of a signed License Agreement or in the absence of this agreement of identifiers allowing to identify the identity of the author, the editors have no right to work with the manuscript.
It is important to remember that there is another type of agreement between authors and publishers – when copyright is transferred from the authors to the publisher. In this case, the authors lose ownership of their work and may not use it in any way.