Assessing the possibility of using a variable-length launch vehicle with a polymer body for orbiting payload
DOI:
https://doi.org/10.15587/1729-4061.2024.306225Keywords:
launch vehicle, variable length, polymer body, aerodynamic characteristics, suborbital trajectoryAbstract
The object of this study was the motion of an ultralight class variable-length launch vehicle made of a polymer body along the active phase of the trajectory. The work considers the solution to the problem of designing low-cost means of delivery to orbit, namely to the assessment of the possibility of removing the payload by a carrier rocket with a polymer body of variable length beyond the dense atmosphere of the Earth. For this purpose, ballistic projection of the trajectory of the launch vehicle was carried out taking into account overloading; its aerodynamic characteristics and peculiarities of aerothermodynamic processes occurring during flight in the atmospheric phase of the trajectory were determined. The closeness (up to 10 %) of the obtained results with known experimental data is shown. The influence of the aerodynamic force on the parameters of the launch vehicle motion was studied. A flight simulation was conducted, the results of which showed the fundamental possibility of launching a CubeSat 24U class payload using a launch vehicle with a polymer body of variable length to a suborbital trajectory with an altitude of about 300 km. At the same time, the effective longitudinal overload on the body of the launch vehicle does not exceed 4 units, and the temperature on the surface of the body does not exceed 300 K. A feature of the research is the use of a multidisciplinary approach, which implies taking into account the interrelationship of aerodynamic, thermodynamic, and ballistic processes. The established motion parameters, aerodynamic characteristics, and the surface heating temperature of the launch vehicle body are key values for further research on the design and analysis of a launch vehicle with a polymer body of variable length. These data could be used to calculate the mechanical and thermal loads acting on the structure of the launch vehicle during flight
References
- Yemets, V., Sanin, F., Dzhur, Y., Masliany, M., Kostritsyn, O., Minteev, G. (2009). Single-stage small satellite launcher with combustible tank of polyethylene. Acta Astronautica, 64 (1), 28–32. https://doi.org/10.1016/j.actaastro.2008.06.015
- Yemets, V., Sanin, F., Masliany, M., Kostritsyn, O., Minteev, G. (2010). Is the combustible inertial pico launch vehicle feasible? JBIS - Journal of the British Interplanetary Society, 63 (7), 249–259.
- Yemets, V., Harkness, P., Dron’, M., Pashkov, A., Worrall, K., Middleton, M. (2018). Autophage Engines: Toward a Throttleable Solid Motor. Journal of Spacecraft and Rockets, 55 (4), 984–992. https://doi.org/10.2514/1.a34153
- Dron, M. M., Dubovyk, L. H., Holubek, O. V., Dreus, A. Yu., Yemets, A. V., Pashkov, A. V. (2019). Systemy vidvodu kosmichnykh obiektiv z nyzkykh navkolozemnykh orbit. Dnipro: LIRA, 218.
- Yemets, V., Dron, M., Pashkov, A., Dreus, A., Kositsyna, Ye., Yemets, M. et al. (2020). Method to preset G-load profile of launch vehicles. Proceedings of the International Astronautical Congress, IAC.
- Yemets, V., Dron’, M., Pashkov, A. (2020). Autophage Engines: Method to Preset Gravity Load of Solid Rockets. Journal of Spacecraft and Rockets, 57 (2), 309–318. https://doi.org/10.2514/1.a34597
- Yemets, V., Dron, M., Dreus, A., Pashkov, A., Yemets, M. (2021). Heat flows in the gasification chamber of the polymer propelled autophage launch vehicle. Proceedings of the International Astronautical Congress.
- Dreus, A., Yemets, V., Dron, M., Yemets, M., Golubek, A. (2021). A simulation of the thermal environment of a plastic body of a new type of launch vehicle at the atmospheric phase of the trajectory. Aircraft Engineering and Aerospace Technology, 94 (4), 505–514. https://doi.org/10.1108/aeat-04-2021-0100
- Dreus, А. Yu., Dron, М. М., Dubovik, L. G., Strembovsky, V. V. (2023). Assessment of the possibility of using polymers in the bodies of promising launch vehicles based on the heat resistance factor. Space Science and Technology, 29 (6), 03–12. https://doi.org/10.15407/knit2023.06.003
- Kondratiev, A. V. (2020). A concept of optimization of structural and technological parameters of polymer composite rocket units considering the character of their production. Space Science and Technology, 26 (6), 5–22. https://doi.org/10.15407/knit2020.06.005
- Kondratiev, A. V., Kovalenko, V. O. (2019). Optimization of design parameters of the main composite fairing of the launch vehicle under simultaneous force and thermal loading. Science and Technology, 25 (4), 3–21. https://doi.org/10.15407/knit2019.04.003
- Kondratiev, A., Potapov, O., Tsaritsynskyi, A., Nabokina, T. (2021). Optimal Design of Composite Shelled Sandwich Structures with a Honeycomb Filler. Advances in Design, Simulation and Manufacturing IV, 546–555. https://doi.org/10.1007/978-3-030-77719-7_54
- Kositsyna, O. S., Dron’, M. M., Yemets, V. V. (2020). The environmental impact assessment of emission from space launches: the promising propellants components selection. Journal of Chemistry and Technologies, 28 (2), 186–193. https://doi.org/10.15421/082020
- Kositsyna, O., Varlan, K., Dron, M., Kulyk, O. (2021). Determining energetic characteristics and selecting environmentally friendly components for solid rocket propellants at the early stages of design. Eastern-European Journal of Enterprise Technologies, 6 (6 (114)), 6–14. https://doi.org/10.15587/1729-4061.2021.247233
- Wang, L., He, G. Y., Wang, Q., Chen, L. S. (2020). An Engineering Method for Computing the Aerodynamics Performance of Hypersonic Vehicle. IOP Conference Series: Materials Science and Engineering, 816 (1), 012006. https://doi.org/10.1088/1757-899x/816/1/012006
- Yang, Z., Wang, S., Gao, Z. (2022). Studies on effects of wall temperature variation on heat transfer in hypersonic laminar boundary layer. International Journal of Heat and Mass Transfer, 190, 122790. https://doi.org/10.1016/j.ijheatmasstransfer.2022.122790
- Zhao, M. (2021). Prediction and Validation Technologies of Aerodynamic Force and Heat for Hypersonic Vehicle Design. In Springer Aerospace Technology. Springer Singapore. https://doi.org/10.1007/978-981-33-6526-1
- Dillenius, M. F. E., Nielsen, J. N. (1979). Computer Programs for Calculating Pressure Distributions Including Vortex Effects on Supersonic Monoplane or Cruciform Wing-Body-Tail Combinations with Round or Elliptical Bodies. NASA. Available at: https://ntrs.nasa.gov/citations/19810018506
- Cengizci, S., Uğur, Ö., Takizawa, K., Tezduyar, T. (2020). A Streamline-Upwind/Petrov-Galerkin Formulation For Supersonic and Hypersonic Flow Simulations. The 20th Biennial Computational Techniques and Applications Conference (CTAC2020). Available at: http://hdl.handle.net/11511/94032
- Li, J., Jiang, D., Geng, X., Chen, J. (2021). Kinetic comparative study on aerodynamic characteristics of hypersonic reentry vehicle from near-continuous flow to free molecular flow. Advances in Aerodynamics, 3 (1). https://doi.org/10.1186/s42774-021-00063-0
- Huang, T., He, G., Wang, Q. (2022). Calculation of Aerodynamic Characteristics of Hypersonic Vehicles Based on the Surface Element Method. Advances in Aerospace Science and Technology, 07 (02), 112–122. https://doi.org/10.4236/aast.2022.72007
- Furudate, M. A. (2022). MC-New: A Program to Calculate Newtonian Aerodynamic Coefficients Based on Monte-Carlo Integration. Aerospace, 9 (6), 330. https://doi.org/10.3390/aerospace9060330
- Thomas, P. D., Vinokur, M., Bastianon, R. A., Conti, R. J. (1972). Numerical Solution for Three-Dimensional Inviscid Supersonic Flow. AIAA Journal, 10 (7), 887–894. https://doi.org/10.2514/3.50241
- Hussein, A. K, Khan, W. A., Sivasankaran, S., Mohammed, H. A., Adegun, I. K. (2013). Numerical simulation of three-dimensional supersonic flow around an aerodynamic bump. Journal of Basic and Applied Scientific Research, 3 (7), 656–666.
- Marchenay, Y., Olazabal Loumé, M., Chedevergne, F. (2022). Hypersonic Turbulent Flow Reynolds-Averaged Navier–Stokes Simulations with Roughness and Blowing Effects. Journal of Spacecraft and Rockets, 59 (5), 1686–1696. https://doi.org/10.2514/1.a35339
- Sofair, I. (1995). Improved Method for Calculating Exact Geodetic Latitude and Altitude. Defense Technical Information Center. https://doi.org/10.21236/ada294568
- Bin, Y., Huang, G., Kunz, R., Yang, X. I. A. (2024). Constrained Recalibration of Reynolds-Averaged Navier–Stokes Models. AIAA Journal, 62 (4), 1434–1446. https://doi.org/10.2514/1.j063407
- Prihod'ko, A. A. (2008). Komp'yuternye tehnologii v aerogidrodinamike i teplomassoobmene. Kyiv: NAUKOVA DUMKA, 380.
- Prykhodko, O. A., Alekseyenko, S. V. (2014). Numerical simulation of the process of airfoil icing in the presence of large supercooled water drops. Technical Physics Letters, 40 (10), 864–867. https://doi.org/10.1134/s1063785014100125
- Prikhod’ko, A. A., Alekseenko, S. V. (2014). Numerical Simulation of the Processes of Icing on Airfoils with Formation of a “Barrier” Ice. Journal of Engineering Physics and Thermophysics, 87 (3), 598–607. https://doi.org/10.1007/s10891-014-1050-0
- Alekseyenko, S., Dreus, A., Dron, M., Brazaluk, O. (2022). Numerical Study of Aerodynamic Characteristics of a Pointed Plate of Variable Elongation in Subsonic and Supersonic Gas Flow. Journal of Advanced Research in Fluid Mechanics and Thermal Sciences, 96 (2), 88–97. https://doi.org/10.37934/arfmts.96.2.8897
- Gutiérrez, R., Llorente, E., Ragni, D., Aranguren, P. (2022). Study on k−ω−Shear Stress Transport Corrections Applied to Airfoil Leading-Edge Roughness Under RANS Framework. Journal of Fluids Engineering, 144 (4). https://doi.org/10.1115/1.4052925
- Adanta, D., Fattah, I. M. R., Muhammad, N. M. (2020). Comparison of standard k-epsilon and SST k-omega turbulence model for breastshot waterwheel simulation. Journal of Mechanical Science and Engineering, 7 (2), 039–044. https://doi.org/10.36706/jmse.v7i2.44
- Costa, L. M. F., Montiel, J. E. S., Corrêa, L., Lofrano, F. C., Nakao, O. S., Kurokawa, F. A. (2022). Influence of standard k-ε, SST κ-ω and LES turbulence models on the numerical assessment of a suspension bridge deck aerodynamic behavior. Journal of the Brazilian Society of Mechanical Sciences and Engineering, 44 (8). https://doi.org/10.1007/s40430-022-03653-1
- Maurício Araújo, A., Antônio Coutinho Silva, A., Leal dos Santos, C., Corte Real Fernandes, E., Menezes, E., José Arruda Moura Rocha, G. et al. (2017). An assessment of different turbulence models on a CFD simulation of air flow past a S814 airfoil. Procceedings of the 24th ABCM International Congress of Mechanicl Engineering. https://doi.org/10.26678/abcm.cobem2017.cob17-0306
- Robinson, D., Hassan, H. (1996). A two-equation turbulence closure model for wall bounded and free shear flows. Fluid Dynamics Conference. https://doi.org/10.2514/6.1996-2057
- Petrov, K. P. (1998). Aerodinamika tel prosteyshih form. Moscow: Fizmatlit, 428.
- Meng, Y., Yan, L., Huang, W., Tong, X. (2020). Numerical Investigation of the Aerodynamic Characteristics of a Missile. IOP Conference Series: Materials Science and Engineering, 887 (1), 012001. https://doi.org/10.1088/1757-899x/887/1/012001
- Bayley, D. J., Hartfield, R. J., Burkhalter, J. E., Jenkins, R. M. (2008). Design Optimization of a Space Launch Vehicle Using a Genetic Algorithm. Journal of Spacecraft and Rockets, 45 (4), 733–740. https://doi.org/10.2514/1.35318
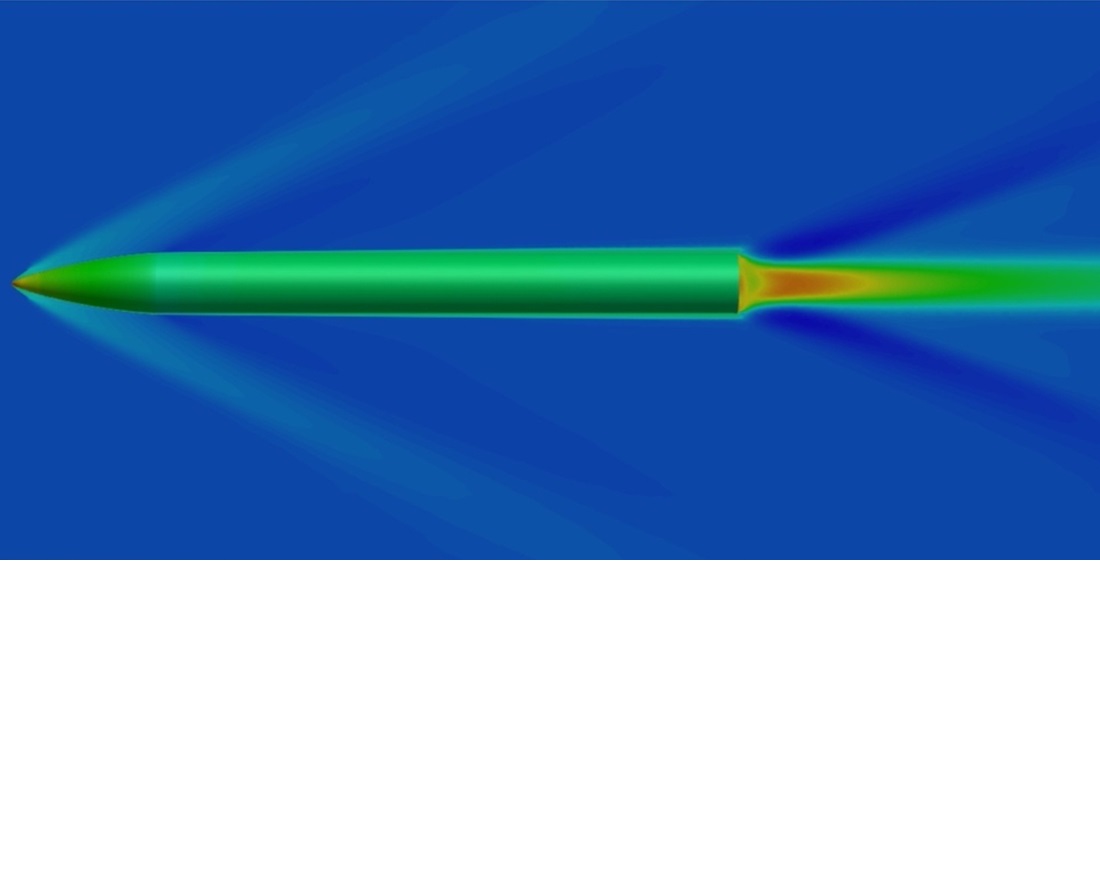
Downloads
Published
How to Cite
Issue
Section
License
Copyright (c) 2024 Aleksandr Golubek, Serhii Aleksieienko, Mykola Dron, Andrii Dreus

This work is licensed under a Creative Commons Attribution 4.0 International License.
The consolidation and conditions for the transfer of copyright (identification of authorship) is carried out in the License Agreement. In particular, the authors reserve the right to the authorship of their manuscript and transfer the first publication of this work to the journal under the terms of the Creative Commons CC BY license. At the same time, they have the right to conclude on their own additional agreements concerning the non-exclusive distribution of the work in the form in which it was published by this journal, but provided that the link to the first publication of the article in this journal is preserved.
A license agreement is a document in which the author warrants that he/she owns all copyright for the work (manuscript, article, etc.).
The authors, signing the License Agreement with TECHNOLOGY CENTER PC, have all rights to the further use of their work, provided that they link to our edition in which the work was published.
According to the terms of the License Agreement, the Publisher TECHNOLOGY CENTER PC does not take away your copyrights and receives permission from the authors to use and dissemination of the publication through the world's scientific resources (own electronic resources, scientometric databases, repositories, libraries, etc.).
In the absence of a signed License Agreement or in the absence of this agreement of identifiers allowing to identify the identity of the author, the editors have no right to work with the manuscript.
It is important to remember that there is another type of agreement between authors and publishers – when copyright is transferred from the authors to the publisher. In this case, the authors lose ownership of their work and may not use it in any way.