Створення багатоконтурної системи безпеки інформаційних взаємодій у соціокиберфізичних системах
DOI:
https://doi.org/10.15587/1729-4061.2023.289467Ключові слова:
соціокіберфізична система, модель безпеки інформаційних взаємодій, соціальна інженерія, цільові атакиАнотація
Об’єктом дослідження є многоконтурная система безпеки інформаційних взаємодій у соціокіберфізичних системах. Динамічний характер фізичних середовищ за своєю природою ставить під сумнів здатність соціокіберфізичних систем виконувати адекватні дії з управління керованими фізичними активами у багатьох контекстах. Однак дії з адаптації та еволюції повинні бути оцінені до їх реалізації в керованій системі, щоб забезпечити стійкість до відмови при мінімізації ризиків. Отже, дизайн соціокіберфізичних систем повинен забезпечувати не тільки надійну автономність, а й експлуатаційну стійкість до відмов і безпеку. Пропонований підхід ґрунтується на комплексуванні цільових (змішаних) загроз на основі синтезу технічних кіберзагроз із методами соціальної інженерії. Такий підхід забезпечує можливість формувати динамічну модель безпеки на основі аналізу взаємодії різних агентів у соціокіберфізичних системах, що дозволяє підвищити рівень протидії цільовим (змішаним) кіберзагрозам.
Наведені результати моделювання ґрунтуються на запропонованій класифікації загроз методів соціальної інженерії, що дозволяє кіберзловмисникам забезпечити ймовірність реалізації цільових загроз до 95–98 %. Запропонована класифікація загроз на основі методів соціальної інженерії дозволить сформувати додатковий параметр об'єктивності цільових загроз з урахуванням їхнього комплексування та синергії. При цьому представлена модель дозволить своєчасно забезпечити знання про можливість реалізації цільової атаки та своєчасно вжити превентивних заходів протидії. Такий підхід дозволить покращити комплекс заходів захисту, а також своєчасно сформувати підвищення рівня протидії персоналу компанії (організації, підприємства тощо) загрозам соціальної інженерії
Посилання
- Graf, S., Quinton, S., Girault, A., Gössler, G. (2018). Building Correct Cyber-Physical Systems: Why We Need a Multiview Contract Theory. Lecture Notes in Computer Science, 19–31. doi: https://doi.org/10.1007/978-3-030-00244-2_2
- Bereket Abera, Y., Naudet, Y., Panetto, H. (2020). A new Paradigm and Meta-Model for Cyber-Physical-Social Systems. IFAC-PapersOnLine, 53 (2), 10949–10954. doi: https://doi.org/10.1016/j.ifacol.2020.12.2841
- Yilma, B. A., Naudet, Y., Panetto, H. (2019). Introduction to Personalisation in Cyber-Physical-Social Systems. Lecture Notes in Computer Science, 25–35. doi: https://doi.org/10.1007/978-3-030-11683-5_3
- Yilma, B. A., Panetto, H., Naudet, Y. (2019). A Meta-Model of Cyber-Physical-Social System: The CPSS Paradigm to Support Human-Machine Collaboration in Industry 4.0. IFIP Advances in Information and Communication Technology, 11–20. doi: https://doi.org/10.1007/978-3-030-28464-0_2
- Naudet, Y., Yilma, B. A., Panetto, H. (2018). Personalisation in Cyber Physical and Social Systems: the Case of Recommendations in Cultural Heritage Spaces. 2018 13th International Workshop on Semantic and Social Media Adaptation and Personalization (SMAP). doi: https://doi.org/10.1109/smap.2018.8501890
- Zeng, J., Yang, L. T., Lin, M., Ning, H., Ma, J. (2020). A survey: Cyber-physical-social systems and their system-level design methodology. Future Generation Computer Systems, 105, 1028–1042. doi: https://doi.org/10.1016/j.future.2016.06.034
- Sheth, A., Anantharam, P., Henson, C. (2013). Physical-Cyber-Social Computing: An Early 21st Century Approach. IEEE Intelligent Systems, 28 (1), 78–82. doi: https://doi.org/10.1109/mis.2013.20
- Wang, F.-Y. (2010). The Emergence of Intelligent Enterprises: From CPS to CPSS. IEEE Intelligent Systems, 25 (4), 85–88. doi: https://doi.org/10.1109/mis.2010.104
- Dzheniuk, N., Yevseiev, S., Opirskyy, I., Voropay, N., Korolev, R., Sydorenko, Z. (2023). Sociocyberphysical System Wireless Air Network Topology Synthesis Model. Mizhnarodnyi naukovo-praktychnyi forum «Tsyfrova realnist». Kiberbezpeka ta informatsiyni tekhnolohiyi v umovakh hibrydnykh viyn. Kharkiv-Odesa, 4–10.
- Horváth, I., Rusák, Z., Li, Y. (2017). Order Beyond Chaos: Introducing the Notion of Generation to Characterize the Continuously Evolving Implementations of Cyber-Physical Systems. Volume 1: 37th Computers and Information in Engineering Conference. doi: https://doi.org/10.1115/detc2017-67082
- Tanik, U. J., Begley, A. (2013). An Adaptive Cyber-Physical System Framework for Cyber-Physical Systems Design Automation. Applied Cyber-Physical Systems, 125–140. doi: https://doi.org/10.1007/978-1-4614-7336-7_11
- Yin, D., Ming, X., Zhang, X. (2020). Understanding Data-Driven Cyber-Physical-Social System (D-CPSS) Using a 7C Framework in Social Manufacturing Context. Sensors, 20 (18), 5319. doi: https://doi.org/10.3390/s20185319
- Hao, K. (2020). OpenAI is giving Microsoft exclusive access to its GPT-3 language model. MIT Technology Review. Available at: https://www.technologyreview.com/2020/09/23/1008729/openai-is-giving-microsoft-exclusive-access-to-its-gpt-3-language-model/
- Goldstein, J. A., Sastry, G., Musser, M., DiResta, R., Gentzel, M., Sedova. K. (2023). Generative Language Models and Automated Influence Operations: Emerging Threats and Potential Mitigations. Available at: https://cdn.openai.com/papers/forecasting-misuse.pdf
- Tabassi, E. (2023). Artificial Intelligence Risk Management Framework. NIST AI 100-1. NIST. doi: https://doi.org/10.6028/nist.ai.100-1
- Wang, Z., Sun, L., Zhu, H. (2020). Defining Social Engineering in Cybersecurity. IEEE Access, 8, 85094–85115. doi: https://doi.org/10.1109/access.2020.2992807
- NIST Special Publication 1270. Proposes a framework for identifying and managing bias in artificial intelligence.
- Foster, D. (2023). Generative Deep Learning. O'Reilly Media, Inc.
- Yevseiev, S., Milevskyi, S., Bortnik, L., Alexey, V., Bondarenko, K., Pohasii, S. (2022). Socio-Cyber-Physical Systems Security Concept. 2022 International Congress on Human-Computer Interaction, Optimization and Robotic Applications (HORA). doi: https://doi.org/10.1109/hora55278.2022.9799957
- Wang, Z., Zhu, H., Sun, L. (2021). Social Engineering in Cybersecurity: Effect Mechanisms, Human Vulnerabilities and Attack Methods. IEEE Access, 9, 11895–11910. doi: https://doi.org/10.1109/access.2021.3051633
- Prete, E. D., Pera, F., Faramondi, L., Fioravanti, C., Guarino, S., Oliva, G., Setola, R. (2020). Anomaly and Attack Detection in Supervisory Control Networks for Cyber-Physical Systems. Proceedings of the 30th European Safety and Reliability Conference and 15th Probabilistic Safety Assessment and Management Conference. doi: https://doi.org/10.3850/978-981-14-8593-0_4315-cd
- Toublanc, T., Guillet, S., de Lamotte, F., Berruet, P., Lapotre, V. (2017). Using a Virtual Plant to Support the Development of Intelligent Gateway for Sensors/Actuators Security. IFAC-PapersOnLine, 50 (1), 5837–5842. doi: https://doi.org/10.1016/j.ifacol.2017.08.541
- Calefato, F., Lanubile, F., Novielli, N. (2017). A Preliminary Analysis on the Effects of Propensity to Trust in Distributed Software Development. 2017 IEEE 12th International Conference on Global Software Engineering (ICGSE). doi: https://doi.org/10.1109/icgse.2017.1
- Lombardi, M., Vannuccini, S. (2022). Understanding emerging patterns and dynamics through the lenses of the cyber-physical universe. Patterns, 3 (11), 100601. doi: https://doi.org/10.1016/j.patter.2022.100601
- Roy, T., Tariq, A., Dey, S. (2022). A Socio-Technical Approach for Resilient Connected Transportation Systems in Smart Cities. IEEE Transactions on Intelligent Transportation Systems, 23 (6), 5019–5028. doi: https://doi.org/10.1109/tits.2020.3045854
- Hamzaoui, M. A., Julien, N. (2022). Social Cyber-Physical Systems and Digital Twins Networks: A perspective about the future digital twin ecosystems. IFAC-PapersOnLine, 55 (8), 31–36. doi: https://doi.org/10.1016/j.ifacol.2022.08.006
- Li, X., Ye, P., Li, J., Liu, Z., Cao, L., Wang, F.-Y. (2022). From Features Engineering to Scenarios Engineering for Trustworthy AI: I&I, C&C, and V&V. IEEE Intelligent Systems, 37 (4), 18–26. doi: https://doi.org/10.1109/mis.2022.3197950
- Lezoche, M., Panetto, H. (2018). Cyber-Physical Systems, a new formal paradigm to model redundancy and resiliency. Enterprise Information Systems, 14 (8), 1150–1171. doi: https://doi.org/10.1080/17517575.2018.1536807
- Sowe, S. K., Simmon, E., Zettsu, K., de Vaulx, F., Bojanova, I. (2016). Cyber-Physical-Human Systems: Putting People in the Loop. IT Professional, 18 (1), 10–13. doi: https://doi.org/10.1109/mitp.2016.14
- Smirnov, A., Shilov, N., Gusikhin, O. (2017). Cyber-physical-human system for connected car-based e-tourism: Approach and case study scenario. 2017 IEEE Conference on Cognitive and Computational Aspects of Situation Management (CogSIMA). doi: https://doi.org/10.1109/cogsima.2017.7929591
- Kumar, S. A. P., Bhargava, B., Macedo, R., Mani, G. (2017). Securing IoT-Based Cyber-Physical Human Systems against Collaborative Attacks. 2017 IEEE International Congress on Internet of Things (ICIOT). doi: https://doi.org/10.1109/ieee.iciot.2017.11
- Zhu, Y., Tan, Y., Li, R., Luo, X. (2015). Cyber-Physical-Social-Thinking Modeling and Computing for Geological Information Service System. 2015 International Conference on Identification, Information, and Knowledge in the Internet of Things (IIKI). doi: https://doi.org/10.1109/iiki.2015.48
- Kannisto, J., Makitalo, N., Aaltonen, T., Mikkonen, T. (2016). Programming Model Perspective on Security and Privacy of Social Cyber-physical Systems. 2016 IEEE International Conference on Mobile Services (MS). doi: https://doi.org/10.1109/mobserv.2016.23
- Xu, Q., Su, Z., Yu, S. (2018). Green Social CPS Based E-Healthcare Systems to Control the Spread of Infectious Diseases. 2018 IEEE International Conference on Communications (ICC). doi: https://doi.org/10.1109/icc.2018.8422421
- Rose, S., Borchert, O., Mitchell, S., Connelly, S. (2020). Zero Trust Architecture. NIST. doi: https://doi.org/10.6028/nist.sp.800-207
- Yevseiev, S., Tolkachov, M., Shetty, D., Khvostenko, V., Strelnikova, A., Milevskyi, S., Golovashych, S. (2023). The concept of building security of the network with elements of the semiotic approach. ScienceRise, 1, 24–34. doi: https://doi.org/10.21303/2313-8416.2023.002828
- Gilchrist, A. (2016). Industry 4.0. Apress Berkeley, 250. doi: https://doi.org/10.1007/978-1-4842-2047-4
- EFFRA. Factories of the Future: Multi-annual Roadmap for the Contractual PPP under Horizon 2020. European Commission. Available at: https://www.effra.eu/sites/default/files/factories_of_the_future_2020_roadmap.pdf
- Monostori, L. (2014). Cyber-physical Production Systems: Roots, Expectations and R&D Challenges. Procedia CIRP, 17, 9–13. doi: https://doi.org/10.1016/j.procir.2014.03.115
- Uhlemann, T. H.-J., Lehmann, C., Steinhilper, R. (2017). The Digital Twin: Realizing the Cyber-Physical Production System for Industry 4.0. Procedia CIRP, 61, 335–340. doi: https://doi.org/10.1016/j.procir.2016.11.152
- Kang, H. S., Lee, J. Y., Choi, S., Kim, H., Park, J. H., Son, J. Y. et al. (2016). Smart manufacturing: Past research, present findings, and future directions. International Journal of Precision Engineering and Manufacturing-Green Technology, 3 (1), 111–128. doi: https://doi.org/10.1007/s40684-016-0015-5
- Sniderman, B., Mahto, M., Cotteleer, M. J. (2016). Industry 4.0 and Manufacturing Ecosystems – Exploring the World of Connected Enterprises. Deloitte University Press. Available at: https://www2.deloitte.com/content/dam/insights/us/articles/manufacturing-ecosystems-exploring-world-connected-enterprises/DUP_2898_Industry4.0ManufacturingEcosystems.pdf
- Hermann, M., Pentek, T., Otto, B. (2016). Design Principles for Industrie 4.0 Scenarios. 2016 49th Hawaii International Conference on System Sciences (HICSS). doi: https://doi.org/10.1109/hicss.2016.488
- Goldstein, J. A., Sastry, G., Musser, M., DiResta, R., Gentzel, M., Sedova, K. (2023). Forecasting potential misuses of language models for disinformation campaigns—and how to reduce risk. Stanford. Available at: https://cyber.fsi.stanford.edu/io/news/forecasting-potential-misuses-language-models-disinformation-campaigns-and-how-reduce-risk
- Kato, T., Kudo, Y., Miyakoshi, J., Otsuka, J., Saigo, H., Karasawa, K. et al. (2020). Rational Choice Hypothesis as X-point of Utility Function and Norm Function. Applied Economics and Finance, 7 (4), 63. doi: https://doi.org/10.11114/aef.v7i4.4890
- Heath, J. (2008). Following the Rules: Practical Reasoning and Deontic Constraint. Oxford University Press. doi: https://doi.org/10.1093/acprof:oso/9780195370294.001.0001
- Yevseiev, S., Melenti, Y., Voitko, O., Hrebeniuk, V., Korchenko, A., Mykus, S., Milov, O. et al. (2021). Development of a concept for building a critical infrastructure facilities security system. Eastern-European Journal of Enterprise Technologies, 3 (9 (111)), 63–83. doi: https://doi.org/10.15587/1729-4061.2021.233533
- Androshchuk, А., Yevseiev, S., Melenchuk, V., Lemeshko, O., Lemeshko, V. (2020). Improvement of project risk assessment methods of implementation of automated information components of non-commercial organizational and technical systems. EUREKA: Physics and Engineering, 1, 48–55. doi: https://doi.org/10.21303/2461-4262.2020.001131
- Yevseiev, S., Ponomarenko, V., Laptiev, O., Milov, O., Korol, O., Milevskyi, S. et. al.; Yevseiev, S., Ponomarenko, V., Laptiev, O., Milov, O. (Eds.) (2021). Synergy of building cybersecurity systems. Kharkiv: РС ТЕСHNOLOGY СЕNTЕR, 188. doi: https://doi.org/10.15587/978-617-7319-31-2
- Yevseiev, S., Hryshchuk, R., Molodetska, K., Nazarkevych, M., Hrytsyk, V., Milov, O. et. al.; Yevseiev, S., Hryshchuk, R., Molodetska, K., Nazarkevych, M. (Eds.) (2022). Modeling of security systems for critical infrastructure facilities. Kharkiv: РС ТЕСHNOLOGY СЕNTЕR, 196. doi: https://doi.org/10.15587/978-617-7319-57-2
- Yevseiev, S., Khokhlachova, Yu., Ostapov, S., Laptiev, O., Korol, O., Milevskyi, S. et. al.; Yevseiev, S., Khokhlachova, Yu., Ostapov, S., Laptiev, O. (Eds.) (2023). Models of socio-cyber-physical systems security. Kharkiv: РС ТЕСHNOLOGY СЕNTЕR, 184. doi: https://doi.org/10.15587/978-617-7319-72-5
- Shmatko, O., Balakireva, S., Vlasov, A., Zagorodna, N., Korol, O., Milov, O. et al. (2020). Development of methodological foundations for designing a classifier of threats to cyberphysical systems. Eastern-European Journal of Enterprise Technologies, 3 (9 (105)), 6–19. doi: https://doi.org/10.15587/1729-4061.2020.205702
- Angiulli, F., Fassetti, F., Serrao, C. (2023). Anomaly detection with correlation laws. Data & Knowledge Engineering, 145, 102181. doi: https://doi.org/10.1016/j.datak.2023.102181
- Bonabeau, E. (2002). Agent-based modeling: Methods and techniques for simulating human systems. Proceedings of the National Academy of Sciences, 99, 7280–7287. doi: https://doi.org/10.1073/pnas.082080899
- Macal, C. M. (2016). Everything you need to know about agent-based modelling and simulation. Journal of Simulation, 10 (2), 144–156. doi: https://doi.org/10.1057/jos.2016.7
- Walker, J. J. (2012). Cyber security concerns for emergency management. Emergency Management. doi: https://doi.org/10.5772/34104
- Ali, N. S. (2016). A four-phase methodology for protecting web applications using an effective real-time technique. International Journal of Internet Technology and Secured Transactions, 6 (4), 303. doi: https://doi.org/10.1504/ijitst.2016.10003854
- Park, K.-J., Zheng, R., Liu, X. (2012). Cyber-physical systems: Milestones and research challenges. Computer Communications, 36 (1), 1–7. doi: https://doi.org/10.1016/j.comcom.2012.09.006
- Hansman, S., Hunt, R. (2005). A taxonomy of network and computer attacks. Computers & Security, 24 (1), 31–43. doi: https://doi.org/10.1016/j.cose.2004.06.011
- Goel, S., Chen, V. (2005). Information security risk analysis – a matrix-based approach. Proceedings of the Information Resource Management Association (IRMA) International Conference. San Diego. Available at: https://www.albany.edu/~GOEL/publications/goelchen2005.pdf
- Kjaerland, M. (2006). A taxonomy and comparison of computer security incidents from the commercial and government sectors. Computers & Security, 25 (7), 522–538. doi: https://doi.org/10.1016/j.cose.2006.08.004
- Blackwell, C. (2010). A security ontology for incident analysis. Proceedings of the Sixth Annual Workshop on Cyber Security and Information Intelligence Research. doi: https://doi.org/10.1145/1852666.1852717
- Ahmad, R., Yunos, Z. (2012). A dynamic cyber terrorism framework. International Journal of Computer Science and Information Security, 10 (2), 149–158.
- Judіn, O. K. (2015). Derzhavnі іnformacіynі resursi [State information resources]. Metodologіya pobudovi klasifіkatora zagroz. Kyiv: NAU, 214.
- Sznajd-Weron, K., Sznajd, J. (2000). Opinion evolution in closed community. International Journal of Modern Physics C, 11 (06), 1157–1165. doi: https://doi.org/10.1142/s0129183100000936
- Deffuant, G., Neau, D., Amblard, F., Weisbuch, G. (2000). Mixing beliefs among interacting agents. Advances in Complex Systems, 03, 87–98. doi: https://doi.org/10.1142/s0219525900000078
- Hegselmann, R., Krause, U. (2002). Opinion dynamics and bounded confidence: Models, analysis and simulation. Journal of Artificial Societies and Social Simulation, 5 (3). Available at: https://www.jasss.org/5/3/2.html
- Weimer, C. W., Miller, J. O., Hill, R. R. (2016). Agent-based modeling: An introduction and primer. 2016 Winter Simulation Conference (WSC). doi: https://doi.org/10.1109/wsc.2016.7822080
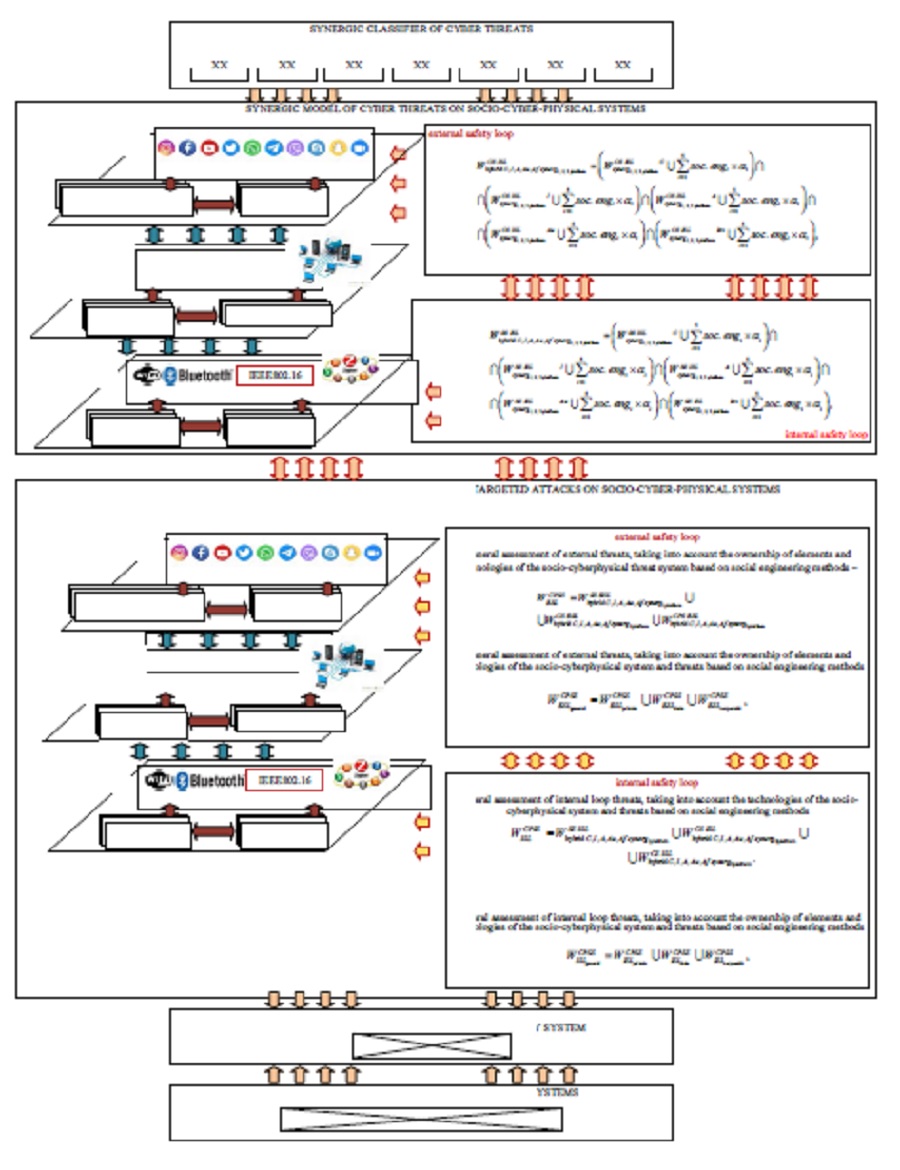
##submission.downloads##
Опубліковано
Як цитувати
Номер
Розділ
Ліцензія
Авторське право (c) 2023 Serhii Yevseiev, Nataliia Dzheniuk, Maksym Tolkachov, Oleksandr Milov, Tetiana Voitko, Mykhailo Prygara, Oleksandr Shpak, Natalia Voropay, Andrii Volkov, Oleksandr Lezik

Ця робота ліцензується відповідно до Creative Commons Attribution 4.0 International License.
Закріплення та умови передачі авторських прав (ідентифікація авторства) здійснюється у Ліцензійному договорі. Зокрема, автори залишають за собою право на авторство свого рукопису та передають журналу право першої публікації цієї роботи на умовах ліцензії Creative Commons CC BY. При цьому вони мають право укладати самостійно додаткові угоди, що стосуються неексклюзивного поширення роботи у тому вигляді, в якому вона була опублікована цим журналом, але за умови збереження посилання на першу публікацію статті в цьому журналі.
Ліцензійний договір – це документ, в якому автор гарантує, що володіє усіма авторськими правами на твір (рукопис, статтю, тощо).
Автори, підписуючи Ліцензійний договір з ПП «ТЕХНОЛОГІЧНИЙ ЦЕНТР», мають усі права на подальше використання свого твору за умови посилання на наше видання, в якому твір опублікований. Відповідно до умов Ліцензійного договору, Видавець ПП «ТЕХНОЛОГІЧНИЙ ЦЕНТР» не забирає ваші авторські права та отримує від авторів дозвіл на використання та розповсюдження публікації через світові наукові ресурси (власні електронні ресурси, наукометричні бази даних, репозитарії, бібліотеки тощо).
За відсутності підписаного Ліцензійного договору або за відсутністю вказаних в цьому договорі ідентифікаторів, що дають змогу ідентифікувати особу автора, редакція не має права працювати з рукописом.
Важливо пам’ятати, що існує і інший тип угоди між авторами та видавцями – коли авторські права передаються від авторів до видавця. В такому разі автори втрачають права власності на свій твір та не можуть його використовувати в будь-який спосіб.