Покращення роздільної здатності та чутливості ортогонального часопролітного мас-спектрометра з ортогональною інжекцією іонів
DOI:
https://doi.org/10.15587/1729-4061.2023.290649Ключові слова:
часопролітний мас-спектрометр, циліндрична імерсійна лінза, трансаксіальне електронне дзеркало, генератор імпульсів, час обертанняАнотація
Розглянуто теоретичні можливості підвищення роздільної здатності та чутливості часопролітного мас-спектрометра з ортогональною інжекцією іонів. Ефекти досягаються за рахунок використання неоднорідних електростатичних полів спеціальної конфігурації як у прискорювальній, так і в фокусуючій частинах приладу – циліндричному імерсійному об’єктиві та трансаксіальному дзеркалі відповідно. Показано, що використання неоднорідного циліндричного поля спеціальної конфігурації як прискорювача іонів відкриває можливість багаторазового зменшення енергетичного розкиду іонів в пакетах інжектованих іонів, пов’язаного з так званим «часом обертання» і , отже, значне (в два і більше разів) збільшення граничної роздільної здатності мас-спектрометра. Використання трансаксіального електростатичного дзеркала як часопролітного мас-аналізатора дозволяє значно підвищити чутливість мас-спектрометра за рахунок реалізації потрійного просторово-часопролітного фокусування пакетів іонів. Основні особливості включають зменшений розкид енергії іонів, підвищену максимальну роздільну здатність і покращену чутливість завдяки потрійному фокусуванню в просторі та часі польоту. Це дослідження закладає основу для розширення можливостей часопролітної мас-спектрометрії, надаючи більш ефективний і потужний інструмент для широкого спектру наукових і промислових застосувань. Ефекти досягаються за рахунок використання неоднорідних електростатичних полів спеціальної конфігурації як в прискорювальній, так і в фокусуючій частинах приладу – циліндричної імерсійної лінзи та трансаксіального дзеркала відповідно. Наведено чисельні розрахунки системи – чотириелектродної циліндричної імерсійної лінзи в поєднанні з триелектродним трансаксіальним дзеркалом, які підтверджують висновки теорії
Посилання
- Chen, Y. H., Gonin, M., Fuhrer, K., Dodonov, A., Su, C. S., Wollnik, H. (1999). Orthogonal electron impact source for a time-of-flight mass spectrometer with high mass resolving power. International Journal of Mass Spectrometry, 185-187, 221–226. doi: https://doi.org/10.1016/s1387-3806(98)14152-0
- Pomozov, T. V., Yavor, M. I., Verentchikov, A. N. (2012). Reflectrons with ion orthogonal acceleration based on planar gridless mirrors. Technical Physics, 57 (4), 550–555. doi: https://doi.org/10.1134/s106378421204024x
- Bimurzaev, S. B. (2019). Planar multi-reflecting time-of-flight mass-spectrometer of a simple design. Advances in Imaging and Electron Physics, 3–13. doi: https://doi.org/10.1016/bs.aiep.2019.08.001
- Mamyrin, B. A. (2001). Time-of-flight mass spectrometry (concepts, achievements, and prospects). International Journal of Mass Spectrometry, 206 (3), 251–266. doi: https://doi.org/10.1016/s1387-3806(00)00392-4
- Golikov, Yu. K., Krasnov, N. V., Bublyaev, R. A., Turtia, S. B., Belyaev, K. A. (2008). Monopole as an orthogonal accelerator TOF analyzer. Nauchnoje Priborostroenie, 18 (4), 97–103.
- Bimurzaev, S. B., Bimurzaeva, R. S., Sarkeev, B. T. (1991). Spatial-TOF focusing in an electrostatic lens-mirror system with two planes of symmetry. Radiotekhnika I Elektronika, 36, 2186–2195.
- Yakushev, E. M., Sekunova, L. M. (1986). Theory of Electron Mirrors and Cathode Lenses. Advances in Electronics and Electron Physics, 337–416. doi: https://doi.org/10.1016/s0065-2539(08)60856-2
- Nevinnyi, Yu. A., Sekunova, L. M., Yakushev, E. M. (1985). Transaxial lens systems for electrostatic prism spectrometers with improved focusing. Zhurnal Tekhnicheskoi Fiziki, 55 (9), 1713–1718.
- Bimurzaev, S. B. (2015). A TOF mass spectrometer with higher resolution and sensitivity via elimination of chromatic TOF aberrations of higher orders. International Journal of Mass Spectrometry, 376, 23–26. doi: https://doi.org/10.1016/j.ijms.2014.11.007
- Bimurzaev, S. B., Aldiyarov, N. U. (2014). Time-of-Flight Mass Spectrometer with Transaxial Ion Reflector. Journal of Modern Physics, 05 (01), 68–73. doi: https://doi.org/10.4236/jmp.2014.51011
- Yakushev, E. M. (2013). Theory and Computation of Electron Mirrors. Advances in Imaging and Electron Physics, 147–247. doi: https://doi.org/10.1016/b978-0-12-407701-0.00003-0
- Karetskaya, S. P., Saichenko, N. Yu. (1989). Four-electrode mirror with a two-dimensional electric field. Zhurnal Tekhnicheskoi Fiziki, 59 (10), 98–103.
- Utegenova, A., Bapyshev, A., Suimenbayeva, Z., Aden, A., Kassym, R., Tansaule, S. (2023). Development system for coordination of activities of experts in the formation of machineschetable standards in the field of military and space activities based on ontological engineering: a case study. Eastern-European Journal of Enterprise Technologies, 5 (2 (125)), 67–77. doi: https://doi.org/10.15587/1729-4061.2023.288542
- Tikkisetty, K., Filewood, T., Yan, J., Kwok, H., Brunswick, P., Cody, R., Shang, D. (2023). Method development for forensic oil identification by direct analysis in real time time-of-flight mass spectrometry. Analytical Methods, 15 (44), 6040–6047. doi: https://doi.org/10.1039/d3ay01282d
- Baibolov, A., Sydykov, S., Alibek, N., Tokmoldayev, A., Turdybek, B., Jurado, F., Kassym, R. (2022). Map of zoning of the territory of Kazakhstan by the average temperature of the heating period in order to select a heat pump system of heat supply: A case study. Energy Sources, Part A: Recovery, Utilization, and Environmental Effects, 44 (3), 7303–7315. doi: https://doi.org/10.1080/15567036.2022.2108168
- Cooper-Shepherd, D. A., Wildgoose, J., Kozlov, B., Johnson, W. J., Tyldesley-Worster, R., Palmer, M. E. et al. (2023). Novel Hybrid Quadrupole-Multireflecting Time-of-Flight Mass Spectrometry System. Journal of the American Society for Mass Spectrometry, 34 (2), 264–272. doi: https://doi.org/10.1021/jasms.2c00281
- Kassym, R. T., Taldybayeva, A. S., Omar, D. R., Alibek, N. B., Kuder, K. M., Isakhanov, M. Z., Omarov, R. A. (2021). Experimental results of functional characteristics of IOT for free range sheep breeding. International Journal of Agricultural Resources, Governance and Ecology, 17 (2/4), 1. doi: https://doi.org/10.1504/ijarge.2021.10044149
- Mohamed, N. A., Hasanien, H. M., Alkuhayli, A., Akmaral, T., Jurado, F., Badr, A. O. (2023). Hybrid Particle Swarm and Gravitational Search Algorithm-Based Optimal Fractional Order PID Control Scheme for Performance Enhancement of Offshore Wind Farms. Sustainability, 15 (15), 11912. doi: https://doi.org/10.3390/su151511912
- Hashish, M. S., Hasanien, H. M., Ji, H., Alkuhayli, A., Alharbi, M., Akmaral, T. et al. (2023). Monte Carlo Simulation and a Clustering Technique for Solving the Probabilistic Optimal Power Flow Problem for Hybrid Renewable Energy Systems. Sustainability, 15 (1), 783. doi: https://doi.org/10.3390/su15010783
- Bimurzaev, S., Sautbekov, S., Sautbekova, Z. (2023). Calculation of the Electrostatic Field of a Circular Cylinder with a Slot by the Wiener–Hopf Method. Mathematics, 11 (13), 2933. doi: https://doi.org/10.3390/math11132933
- Bimurzaev, S. B., Yakushev, E. M. (2022). Relativistic theory of aberrations of electrostatic electron-optical systems. Nuclear Instruments and Methods in Physics Research Section A: Accelerators, Spectrometers, Detectors and Associated Equipment, 1022, 165956. doi: https://doi.org/10.1016/j.nima.2021.165956
- Bimurzaev, S. B., Yakushev, E. M. (2021). Theory and Calculation of Electrostatic Electron Mirrors with Allowance for Relativistic Effects. Technical Physics, 66 (5), 690–698. doi: https://doi.org/10.1134/s1063784221050054
- Vestal, M., Li, L., Dobrinskikh, E., Shi, Y., Wang, B., Shi, X. et al. (2019). Rapid MALDI‐TOF molecular imaging: Instrument enhancements and their practical consequences. Journal of Mass Spectrometry, 55 (8). doi: https://doi.org/10.1002/jms.4423
- Willis, P., Jaloszynski, J., Artaev, V. (2021). Improving duty cycle in the Folded Flight Path high-resolution time-of-flight mass spectrometer. International Journal of Mass Spectrometry, 459, 116467. doi: https://doi.org/10.1016/j.ijms.2020.116467
- Plumb, R. S., McDonald, T., Rainville, P. D., Hill, J., Gethings, L. A., Johnson, K. A., Wilson, I. D. (2021). High-Throughput UHPLC/MS/MS-Based Metabolic Profiling Using a Vacuum Jacketed Column. Analytical Chemistry, 93 (30), 10644–10652. doi: https://doi.org/10.1021/acs.analchem.1c01982
- Amaral, M. S. S., Nolvachai, Y., Marriott, P. J. (2019). Comprehensive Two-Dimensional Gas Chromatography Advances in Technology and Applications: Biennial Update. Analytical Chemistry, 92 (1), 85–104. doi: https://doi.org/10.1021/acs.analchem.9b05412
- Giles, K., Ujma, J., Wildgoose, J., Pringle, S., Richardson, K., Langridge, D., Green, M. (2019). A Cyclic Ion Mobility-Mass Spectrometry System. Analytical Chemistry, 91 (13), 8564–8573. doi: https://doi.org/10.1021/acs.analchem.9b01838
- Yavor, M. I., Verenchikov, A. N., Guluev, R. G. (2019). Cylindrical sector field multi-turn time-of-flight mass analyzer with second order focusing. International Journal of Mass Spectrometry, 442, 58–63. doi: https://doi.org/10.1016/j.ijms.2019.05.007
- Richardson, K., Hoyes, J. (2015). A novel multipass oa-TOF mass spectrometer. International Journal of Mass Spectrometry, 377, 309–315. doi: https://doi.org/10.1016/j.ijms.2014.08.031
- Rose, T., Appleby, R. B., Nixon, P., Richardson, K., Green, M. (2020). Segmented electrostatic trap with inductive, frequency based, mass-to-charge ion determination. International Journal of Mass Spectrometry, 450, 116304. doi: https://doi.org/10.1016/j.ijms.2020.116304
- Buchberger, A. R., DeLaney, K., Johnson, J., Li, L. (2017). Mass Spectrometry Imaging: A Review of Emerging Advancements and Future Insights. Analytical Chemistry, 90 (1), 240–265. doi: https://doi.org/10.1021/acs.analchem.7b04733
- Ferey, J., Larroque, M., Schmitz-Afonso, I., Le Maître, J., Sgarbura, O., Carrere, S. et al. (2022). Imaging Matrix-Assisted Laser Desorption/Ionization Fourier Transform Ion Cyclotron Resonance Mass Spectrometry of oxaliplatin derivatives in human tissue sections. Talanta, 237, 122915. doi: https://doi.org/10.1016/j.talanta.2021.122915
- Vandergrift, G. W., Kew, W., Lukowski, J. K., Bhattacharjee, A., Liyu, A. V., Shank, E. A. et al. (2022). Imaging and Direct Sampling Capabilities of Nanospray Desorption Electrospray Ionization with Absorption-Mode 21 Tesla Fourier Transform Ion Cyclotron Resonance Mass Spectrometry. Analytical Chemistry, 94 (8), 3629–3636. doi: https://doi.org/10.1021/acs.analchem.1c05216
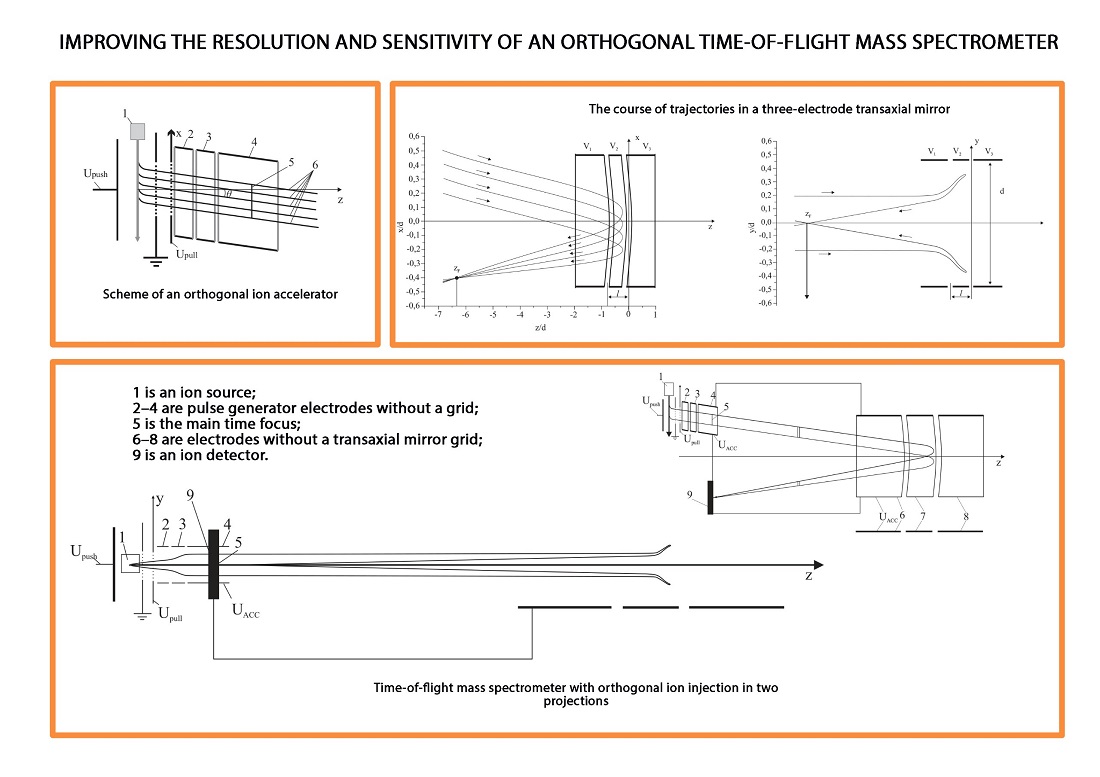
##submission.downloads##
Опубліковано
Як цитувати
Номер
Розділ
Ліцензія
Авторське право (c) 2023 Seitkerim Bimurzaev, Nakhypbek Aldiyarov, Yerkin Yerzhigitov, Akmaral Tlenshiyeva, Ruslan Kassym

Ця робота ліцензується відповідно до Creative Commons Attribution 4.0 International License.
Закріплення та умови передачі авторських прав (ідентифікація авторства) здійснюється у Ліцензійному договорі. Зокрема, автори залишають за собою право на авторство свого рукопису та передають журналу право першої публікації цієї роботи на умовах ліцензії Creative Commons CC BY. При цьому вони мають право укладати самостійно додаткові угоди, що стосуються неексклюзивного поширення роботи у тому вигляді, в якому вона була опублікована цим журналом, але за умови збереження посилання на першу публікацію статті в цьому журналі.
Ліцензійний договір – це документ, в якому автор гарантує, що володіє усіма авторськими правами на твір (рукопис, статтю, тощо).
Автори, підписуючи Ліцензійний договір з ПП «ТЕХНОЛОГІЧНИЙ ЦЕНТР», мають усі права на подальше використання свого твору за умови посилання на наше видання, в якому твір опублікований. Відповідно до умов Ліцензійного договору, Видавець ПП «ТЕХНОЛОГІЧНИЙ ЦЕНТР» не забирає ваші авторські права та отримує від авторів дозвіл на використання та розповсюдження публікації через світові наукові ресурси (власні електронні ресурси, наукометричні бази даних, репозитарії, бібліотеки тощо).
За відсутності підписаного Ліцензійного договору або за відсутністю вказаних в цьому договорі ідентифікаторів, що дають змогу ідентифікувати особу автора, редакція не має права працювати з рукописом.
Важливо пам’ятати, що існує і інший тип угоди між авторами та видавцями – коли авторські права передаються від авторів до видавця. В такому разі автори втрачають права власності на свій твір та не можуть його використовувати в будь-який спосіб.