Розробка моделі механізму подрібнення стиранням в барабанному млині на основі візуалізації даних
DOI:
https://doi.org/10.15587/1729-4061.2024.301653Ключові слова:
барабанний млин, внутрішньокамерне завантаження, подрібнення стиранням, гранулярна температура, продуктивність подрібненняАнотація
Об’єктом дослідження є процес подрібнення в барабанному млині при реалізації механізму руйнування стиранням, який спричинено механізмом навантаження зсуванням. Враховано стираючу дію внаслідок імпульсної взаємодії при взаємному хаотичному переміщенні зернистих частинок у зсувному шарі завантаження, що характеризується гранулярною температурою.
Вирішувалась проблема визначення параметрів зсувної взаємодії, яку викликано труднощами моделювання та складністю апаратурного аналізу поведінки внутрішньомлинного завантаження.
Побудовано математичну модель на основі візуалізації даних для механізму подрібнення стиранням.
Як аналог продуктивності подрібнення прийнято потужність сил зсувної взаємодії. Вихідною характеристикою зсування вважалась усереднене значення градієнта зсувної швидкості у центральному усередненому нормальному перерізі зсувного шару. Враховано вплив на продуктивність гранулярної температури і масової частки зсувного шару та оборотності завантаження.
Експериментальним моделюванням оцінено вплив швидкості обертання на продуктивність при ступені заповнення камери 0.45 та відносному розмірі частинок 0.0104. Встановлено максимальне значення енергії та продуктивності подрібнення стиранням при відносній швидкості обертання ψω=0.55–0.6.
Отримані результати дозволили встановити раціональну швидкість при подрібненні стиранням ψω=0.5–0.6. Це значення є меншим у порівнянні з подрібненням роздавлюванням ψω=0.55–0.65 та розбиванням ψω=0.75–0.9. Встановлений ефект пояснюється виявленою активізацією хаотичного квазітермодинамічного переміщення частинок зсувного шару при тихохідному обертанні.
Розроблена модель дозволяє прогнозувати раціональні технологічні параметри енергоощадного процесу тонкого подрібнення в барабанному млині стиранням
Посилання
- Gupta, V. K. (2020). Energy absorption and specific breakage rate of particles under different operating conditions in dry ball milling. Powder Technology, 361, 827–835. https://doi.org/10.1016/j.powtec.2019.11.033
- Góralczyk, M., Krot, P., Zimroz, R., Ogonowski, S. (2020). Increasing Energy Efficiency and Productivity of the Comminution Process in Tumbling Mills by Indirect Measurements of Internal Dynamics – An Overview. Energies, 13 (24), 6735. https://doi.org/10.3390/en13246735
- Tavares, L. M. (2017). A Review of Advanced Ball Mill Modelling. KONA Powder and Particle Journal, 34, 106–124. https://doi.org/10.14356/kona.2017015
- Semsari Parapari, P., Parian, M., Rosenkranz, J. (2020). Breakage process of mineral processing comminution machines – An approach to liberation. Advanced Powder Technology, 31 (9), 3669–3685. https://doi.org/10.1016/j.apt.2020.08.005
- Napier-Munn, T. J., Morrell, S., Morrison, R. D., Kojovic, T. (1996). Mineral comminution circuits: their operation and optimisation. JKMRC Monograph series in mining and mineral processing, 2.
- Ye, X., Gredelj, S., Skinner, W., Grano, S. R. (2010). Regrinding sulphide minerals — Breakage mechanisms in milling and their influence on surface properties and flotation behaviour. Powder Technology, 203 (2), 133–147. https://doi.org/10.1016/j.powtec.2010.05.002
- Hasan, M., Palaniandy, S., Hilden, M., Powell, M. (2017). Calculating breakage parameters of a batch vertical stirred mill. Minerals Engineering, 111, 229–237. https://doi.org/10.1016/j.mineng.2017.06.024
- Chen, X., Peng, Y., Bradshaw, D. (2014). The effect of particle breakage mechanisms during regrinding on the subsequent cleaner flotation. Minerals Engineering, 66-68, 157–164. https://doi.org/10.1016/j.mineng.2014.04.020
- Wills, B. A., Finch, J. (2015). Wills’ Mineral Processing Technology. Butterworth-Heinemann. https://doi.org/10.1016/c2010-0-65478-2
- Naumenko, Y., Deineka, K. (2023). Building a model of the compression grinding mechanism in a tumbling mill based on data visualization. Eastern-European Journal of Enterprise Technologies, 5 (1 (125)), 64–72. https://doi.org/10.15587/1729-4061.2023.287565
- Naumenko, Y., Deineka, K. (2023). Building a model of the impact grinding mechanism in a tumbling mill based on data visualization. Eastern-European Journal of Enterprise Technologies, 3 (7 (123)), 65–73. https://doi.org/10.15587/1729-4061.2023.283073
- Morrison, A. J., Govender, I., Mainza, A. N., Parker, D. J. (2016). The shape and behaviour of a granular bed in a rotating drum using Eulerian flow fields obtained from PEPT. Chemical Engineering Science, 152, 186–198. https://doi.org/10.1016/j.ces.2016.06.022
- de Klerk, D. N., Govender, I., Mainza, A. N. (2019). Geometric features of tumbling mill flows: A positron emission particle tracking investigation. Chemical Engineering Science, 206, 41–49. https://doi.org/10.1016/j.ces.2019.05.020
- Jaeger, H. M., Nagel, S. R., Behringer, R. P. (1996). Granular solids, liquids, and gases. Reviews of Modern Physics, 68 (4), 1259–1273. https://doi.org/10.1103/revmodphys.68.1259
- Forterre, Y., Pouliquen, O. (2008). Flows of Dense Granular Media. Annual Review of Fluid Mechanics, 40 (1), 1–24. https://doi.org/10.1146/annurev.fluid.40.111406.102142
- Forterre, Y., Pouliquen, O. (2011). Granular Flows. Glasses and Grains, 77–109. https://doi.org/10.1007/978-3-0348-0084-6_4
- Brown, R. L., Richards, J. C. (2016). Principles of powder mechanics: essays on the packing and flow of powders and bulk solids. Elsevier. https://doi.org/10.1016/c2013-0-01576-9
- Savage, S. B. (1984). The Mechanics of Rapid Granular Flows. Advances in Applied Mechanics, 289–366. https://doi.org/10.1016/s0065-2156(08)70047-4
- Campbell, C. (1990). Rapid Granular Flows. Annual Review of Fluid Mechanics, 22 (1), 57–92. https://doi.org/10.1146/annurev.fluid.22.1.57
- Campbell, C. S. (2006). Granular material flows – An overview. Powder Technology, 162 (3), 208–229. https://doi.org/10.1016/j.powtec.2005.12.008
- Jiang, Y., Liu, M. (2009). Granular solid hydrodynamics. Granular Matter, 11 (3), 139–156. https://doi.org/10.1007/s10035-009-0137-3
- Ogawa, S. (1978). Multitemperature theory of granular materials. Proc. of the US-Japan Seminar on Continuum Mechanical and Statistical Approaches in the Mechanics of Granular Materials, 208–217.
- Ogawa, S., Umemura, A., Oshima, N. (1980). On the equations of fully fluidized granular materials. Zeitschrift Für Angewandte Mathematik Und Physik ZAMP, 31 (4), 483–493. https://doi.org/10.1007/bf01590859
- Sun, Q., Song, S., Jin, F., Jiang, Y. (2012). Entropy productions in granular materials. Theoretical and Applied Mechanics Letters, 2 (2), 021002. https://doi.org/10.1063/2.1202102
- Sun, Q., Song, S., Liu, J., Fei, M., Jin, F. (2013). Granular materials: Bridging damaged solids and turbulent fluids. Theoretical and Applied Mechanics Letters, 3 (2), 021008. https://doi.org/10.1063/2.1302108
- Sun, Q., Jin, F., Wang, G., Song, S., Zhang, G. (2015). On granular elasticity. Scientific Reports, 5 (1). https://doi.org/10.1038/srep09652
- Yang, H., Li, R., Kong, P., Sun, Q. C., Biggs, M. J., Zivkovic, V. (2015). Avalanche dynamics of granular materials under the slumping regime in a rotating drum as revealed by speckle visibility spectroscopy. Physical Review E, 91 (4). https://doi.org/10.1103/physreve.91.042206
- Li, R., Yang, H., Zheng, G., Zhang, B. F., Fei, M. L., Sun, Q. C. (2016). Double speckle-visibility spectroscopy for the dynamics of a passive layer in a rotating drum. Powder Technology, 295, 167–174. https://doi.org/10.1016/j.powtec.2016.03.031
- Yang, H., Zhang, B. F., Li, R., Zheng, G., Zivkovic, V. (2017). Particle dynamics in avalanche flow of irregular sand particles in the slumping regime of a rotating drum. Powder Technology, 311, 439–448. https://doi.org/10.1016/j.powtec.2017.01.064
- Li, R., Yang, H., Zheng, G., Sun, Q. C. (2018). Granular avalanches in slumping regime in a 2D rotating drum. Powder Technology, 326, 322–326. https://doi.org/10.1016/j.powtec.2017.12.032
- Yang, H., Zhu, Y., Li, R., Sun, Q. (2020). Kinetic granular temperature and its measurement using speckle visibility spectroscopy. Particuology, 48, 160–169. https://doi.org/10.1016/j.partic.2018.07.011
- Jing, Z., Yang, H., Wang, S., Chen, Q., Li, R. (2021). Comparison of granular temperature measured by SVS and DEM in the rotating cylinder. Powder Technology, 380, 282–287. https://doi.org/10.1016/j.powtec.2020.11.073
- Dolgunin, V. N., Ivanov, O. O., Akopyan, S. A. (2020). Quasithermal Effects During Rapid Gravity Flow of a Granular Medium. Advanced Materials & Technologies, 3 (19), 047–055. https://doi.org/10.17277/amt.2020.03.pp.047-055
- Li, S., Yao, Q., Chen, B., Zhang, X., Ding, Y. L. (2007). Molecular dynamics simulation and continuum modelling of granular surface flow in rotating drums. Chinese Science Bulletin, 52 (5), 692–700. https://doi.org/10.1007/s11434-007-0069-4
- Yin, H., Zhang, M., Liu, H. (2014). Numerical simulation of three-dimensional unsteady granular flows in rotary kiln. Powder Technology, 253, 138–145. https://doi.org/10.1016/j.powtec.2013.10.044
- Yang, S., Sun, Y., Zhang, L., Chew, J. W. (2017). Segregation dynamics of a binary-size mixture in a three-dimensional rotating drum. Chemical Engineering Science, 172, 652–666. https://doi.org/10.1016/j.ces.2017.07.019
- Yang, S., Wang, H., Wei, Y., Hu, J., Chew, J. W. (2020). Flow dynamics of binary mixtures of non-spherical particles in the rolling-regime rotating drum. Powder Technology, 361, 930–942. https://doi.org/10.1016/j.powtec.2019.10.110
- Longo, S., Lamberti, A. (2002). Grain shear flow in a rotating drum. Experiments in Fluids, 32 (3), 313–325. https://doi.org/10.1007/s003480100359
- Chou, H.-T., Lee, C.-F. (2008). Cross-sectional and axial flow characteristics of dry granular material in rotating drums. Granular Matter, 11 (1), 13–32. https://doi.org/10.1007/s10035-008-0118-y
- Chou, S. H., Hsiau, S. S. (2011). Experimental analysis of the dynamic properties of wet granular matter in a rotating drum. Powder Technology, 214 (3), 491–499. https://doi.org/10.1016/j.powtec.2011.09.010
- Chou, S. H., Hu, H. J., Hsiau, S. S. (2016). Investigation of friction effect on granular dynamic behavior in a rotating drum. Advanced Powder Technology, 27 (5), 1912–1921. https://doi.org/10.1016/j.apt.2016.06.022
- Liao, C.-C., Lan, H.-W., Hsiau, S.-S. (2016). Density-induced granular segregation in a slurry rotating drum. International Journal of Multiphase Flow, 84, 1–8. https://doi.org/10.1016/j.ijmultiphaseflow.2016.04.015
- Liao, C.-C. (2019). Effect of dynamic properties on density-driven granular segregation in a rotating drum. Powder Technology, 345, 151–158. https://doi.org/10.1016/j.powtec.2018.12.093
- Liao, C.-C., Ou, S.-F., Chen, S.-L., Chen, Y.-R. (2020). Influences of fine powder on dynamic properties and density segregation in a rotating drum. Advanced Powder Technology, 31 (4), 1702–1707. https://doi.org/10.1016/j.apt.2020.02.006
- Chung, Y.-C., Liao, C.-C., Zhuang, Z.-H. (2021). Experimental investigations for the effect of fine powders on size-induced segregation in binary granular mixtures. Powder Technology, 387, 270–276. https://doi.org/10.1016/j.powtec.2021.04.034
- Govender, I., Richter, M. C., Mainza, A. N., De Klerk, D. N. (2016). A positron emission particle tracking investigation of the scaling law governing free surface flows in tumbling mills. AIChE Journal, 63 (3), 903–913. https://doi.org/10.1002/aic.15453
- Xiu, W., Li, R., Chen, Q., Sun, Q., Zivkovic, V., Yang, H. (2023). Prediction of segregation characterization based on granular velocity and concentration in rotating drum. Particuology, 73, 17–25. https://doi.org/10.1016/j.partic.2022.03.008
- Naumenko, Y. (2017). Modeling a flow pattern of the granular fill in the cross section of a rotating chamber. Eastern-European Journal of Enterprise Technologies, 5 (1 (89)), 59–69. https://doi.org/10.15587/1729-4061.2017.110444
- Naumenko, Y. (2017). Modeling of fracture surface of the quasi solid-body zone of motion of the granular fill in a rotating chamber. Eastern-European Journal of Enterprise Technologies, 2 (1 (86)), 50–57. https://doi.org/10.15587/1729-4061.2017.96447
- Naumenko, Y., Sivko, V. (2017). The rotating chamber granular fill shear layer flow simulation. Eastern-European Journal of Enterprise Technologies, 4 (7 (88)), 57–64. https://doi.org/10.15587/1729-4061.2017.107242
- Deineka, K., Naumenko, Y. (2019). Revealing the effect of decreased energy intensity of grinding in a tumbling mill during self-excitation of auto-oscillations of the intrachamber fill. Eastern-European Journal of Enterprise Technologies, 1 (1 (97)), 6–15. https://doi.org/10.15587/1729-4061.2019.155461
- Deineka, K., Naumenko, Y. (2019). Establishing the effect of a decrease in power intensity of self-oscillating grinding in a tumbling mill with a reduction in an intrachamber fill. Eastern-European Journal of Enterprise Technologies, 6 (7 (102)), 43–52. https://doi.org/10.15587/1729-4061.2019.183291
- Deineka, K., Naumenko, Y. (2020). Establishing the effect of decreased power intensity of self-oscillatory grinding in a tumbling mill when the crushed material content in the intra-chamber fill is reduced. Eastern-European Journal of Enterprise Technologies, 4 (1 (106)), 39–48. https://doi.org/10.15587/1729-4061.2020.209050
- Deineka, K., Naumenko, Y. (2021). Establishing the effect of a simultaneous reduction in the filling load inside a chamber and in the content of the crushed material on the energy intensity of self-oscillatory grinding in a tumbling mill. Eastern-European Journal of Enterprise Technologies, 1 (1 (109)), 77–87. https://doi.org/10.15587/1729-4061.2021.224948
- Deineka, K., Naumenko, Y. (2022). Revealing the mechanism of stability loss of a two-fraction granular flow in a rotating drum. Eastern-European Journal of Enterprise Technologies, 4 (1 (118)), 34–46. https://doi.org/10.15587/1729-4061.2022.263097
- Deineka, K. Yu., Naumenko, Yu. V. (2018). The tumbling mill rotation stability. Scientific Bulletin of National Mining University, 1, 60–68. https://doi.org/10.29202/nvngu/2018-1/10
- Gupta, V. K., Sharma, S. (2014). Analysis of ball mill grinding operation using mill power specific kinetic parameters. Advanced Powder Technology, 25 (2), 625–634. https://doi.org/10.1016/j.apt.2013.10.003
- Hanumanthappa, H., Vardhan, H., Mandela, G. R., Kaza, M., Sah, R., Shanmugam, B. K. (2020). A comparative study on a newly designed ball mill and the conventional ball mill performance with respect to the particle size distribution and recirculating load at the discharge end. Minerals Engineering, 145, 106091. https://doi.org/10.1016/j.mineng.2019.106091
- ISO 924:1989. Coal preparation plant. Principles and conventions for flowsheets. Available at: https://www.iso.org/standard/5340.html
- DIN EN 1009-3. Maschinen für die mechanische Aufbereitung von Mineralien und ähnlichen festen Stoffen - Sicherheit - Teil 3: Spezifische Anforderungen für Brecher und Mühlen; Deutsche Fassung EN 1009-3:2020. Available at: https://www.din.de/de/mitwirken/normenausschuesse/nam/veroeffentlichungen/wdc-beuth:din21:316006092
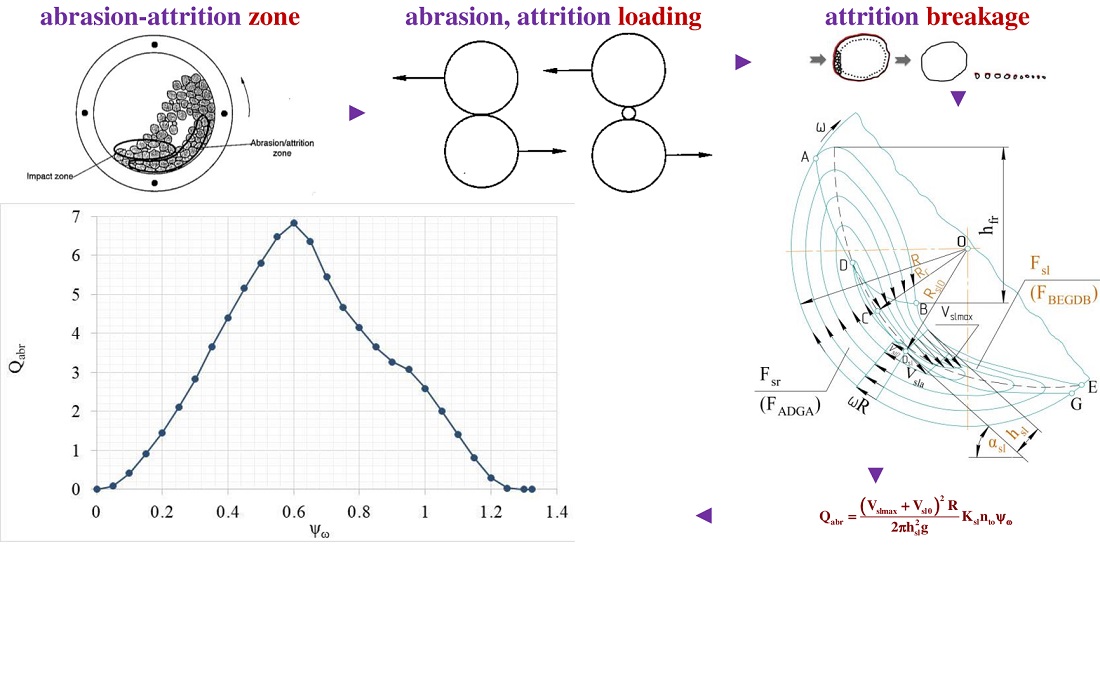
##submission.downloads##
Опубліковано
Як цитувати
Номер
Розділ
Ліцензія
Авторське право (c) 2024 Yuriy Naumenko, Kateryna Deineka, Serhii Zabchyk

Ця робота ліцензується відповідно до Creative Commons Attribution 4.0 International License.
Закріплення та умови передачі авторських прав (ідентифікація авторства) здійснюється у Ліцензійному договорі. Зокрема, автори залишають за собою право на авторство свого рукопису та передають журналу право першої публікації цієї роботи на умовах ліцензії Creative Commons CC BY. При цьому вони мають право укладати самостійно додаткові угоди, що стосуються неексклюзивного поширення роботи у тому вигляді, в якому вона була опублікована цим журналом, але за умови збереження посилання на першу публікацію статті в цьому журналі.
Ліцензійний договір – це документ, в якому автор гарантує, що володіє усіма авторськими правами на твір (рукопис, статтю, тощо).
Автори, підписуючи Ліцензійний договір з ПП «ТЕХНОЛОГІЧНИЙ ЦЕНТР», мають усі права на подальше використання свого твору за умови посилання на наше видання, в якому твір опублікований. Відповідно до умов Ліцензійного договору, Видавець ПП «ТЕХНОЛОГІЧНИЙ ЦЕНТР» не забирає ваші авторські права та отримує від авторів дозвіл на використання та розповсюдження публікації через світові наукові ресурси (власні електронні ресурси, наукометричні бази даних, репозитарії, бібліотеки тощо).
За відсутності підписаного Ліцензійного договору або за відсутністю вказаних в цьому договорі ідентифікаторів, що дають змогу ідентифікувати особу автора, редакція не має права працювати з рукописом.
Важливо пам’ятати, що існує і інший тип угоди між авторами та видавцями – коли авторські права передаються від авторів до видавця. В такому разі автори втрачають права власності на свій твір та не можуть його використовувати в будь-який спосіб.