Substantiating promising technical solutions for turbo- expander power plants based on the research into working processes and states
DOI:
https://doi.org/10.15587/1729-4061.2023.285865Keywords:
discrete-continuous strengthening, contact interaction, stressed-strained state, technical characteristics, turbo-expander, heat and mass transferAbstract
One of the most rational methods of energy utilization of compressed gas in pipelines is to use turbo-expander installations. In particular, these are autonomous turbo-expander power stations. A fundamentally new concept has been devised to improve the technical and economic performance of this type of machines. This concept is not focused on a separate aspect of the plant's operation but on their entire set. In particular, physical principles, structures, and technologies were considered as an object of research. First, effective parameters of gas-dynamic flows and heat-mass transfer were determined based on the modeling of work processes. Secondly, progressive designs of turbo-expander units have been created. Thirdly, technologies for the production of parts and assemblies of turbo-expander units have been developed, which combine, unlike the traditional ones, different types of strengthening for contacting parts in their pair. A method of parametric modeling was used to substantiate the technical solutions of the elements of turbo-expander power plants. This makes it possible to determine the technical characteristics of these installations under a certain set of parameters. By purposeful variation, a recommended set of their parameters was determined, which ensure the improvement of the most important technical characteristics. A specialized database was built, which contains an array of information about the regularities of the influence of variation of significant parameters on various characteristics of turbo-expander power plants. Already on this basis, the problems of synthesis of successful technical solutions of turbo-expander power plants are solved. As a result, their high energy efficiency is ensured. Thus, the efficiency of the expander was achieved at the level of 86 % while the resource increased by 20–25 %. All these solutions were implemented in a number of unique turbo-expander units. Their effectiveness has been demonstrated during operation
References
- Avetian, T., Rodriguez, L. (2020). Fundamentals of turboexpander design and operation. Available at: https://fliphtml5.com/gktj/sepi/basic
- Logan Jr., E. (2003). Handbook of Turbomachinery. CRC Press, 880. doi: https://doi.org/10.1201/9780203911990
- Dixon, S. L., Hall, C. A. (2010). Fluid Mechanics and Thermodynamics of Turbomachinery. Elsevier. doi: https://doi.org/10.1016/c2009-0-20205-4
- Simms, J. (2009). Fundamentals of Turboexpanders “basic theory and design”. Available at: https://www.researchgate.net/file.PostFileLoader.html?id=5236f8abd039b1146f66ab16&assetKey=AS%3A272141839208458%401441895078987
- Saravanamuttoo, H. I. H., Cohen, H., Rogers, G. F. C. (2008). Gas Turbine Theory. Pearson. Available at: https://soaneemrana.org/onewebmedia/GAS%20TURBINE%20THEORY%20BY%20HIH%20SARAVANAMUTTOO,%20H.%20COHEN%20&%20GFC%20ROGERS.pdf
- White, F. (2008). Fluid Mechanics. McGraw-Hill. Available at: http://ftp.demec.ufpr.br/disciplinas/TM240/Marchi/Bibliografia/White_2011_7ed_Fluid-Mechanics.pdf
- Kundu, P., Cohen, I., Dowling, D. (2016). Fluid mechanics. Academic Press. doi: https://doi.org/10.1016/c2012-0-00611-4
- Korpela, S. A. (2011). Principles of Turbomachinery. John Wiley & Sons. doi: https://doi.org/10.1002/9781118162477
- Gorla, R. S. R., Khan, A. A. (2003). Turbomachinery Design and Theory. CRC Press. doi: https://doi.org/10.1201/9780203911600
- Expander-generator. Available at: https://turbogaz.com.ua/uk/equipment/dgu_ua
- Moiseev, A. N. (2015). Energoeffektivnaya turboelektromekhanicheskaya sistema dlya gazoraspredelitel'nykh stantsiy. Visnyk NTU «KhPI», 12 (1121), 356–359. Available at: http://library.kpi.kharkov.ua/files/Vestniki/2015_12_0.pdf
- Kuprygin, O., Moiseev, S., Pastukhova, E., Polivanov, V. (2005). Utilizatsionnye turbodetandernye agregaty OAO «Turbogaz». Energetica Moldovei. Aspecte regionale de dezvoltare. Ediția I. Chișinău, 260–265. Available at: https://ibn.idsi.md/vizualizare_articol/64789
- Fluitech Systems. Available at: http://fluitech.com.ua/
- Marchenko, A., Grabovskiy, A., Tkachuk, M., Shut, O., Tkachuk, M. (2021). Detuning of a Supercharger Rotor from Critical Rotational Velocities. Advances in Design, Lecture Notes in Mechanical Engineering, 137–145. doi: https://doi.org/10.1007/978-3-030-77823-1_14
- Tkachuk, M., Shut, O., Marchenko, A., Grabovskiy, A., Lipeiko, A., Polyvianchuk, A. et al. (2021). Strength and Stability Criteria Limiting Geometrical Dimensions of a Cantilever Impeller. SAE Technical Paper Series. doi: https://doi.org/10.4271/2021-01-5056
- Marchenko, A., Pylyov, V., Linkov, O. (2021). Estimation of Strength of the Combustion Chamber of the ICE Piston with a TBC Layer. Integrated Computer Technologies in Mechanical Engineering - 2020, 415–426. doi: https://doi.org/10.1007/978-3-030-66717-7_35
- Tkachuk, M. M., Grabovskiy, A., Tkachuk, M. A., Zarubina, A., Lipeyko, A. (2021). Analysis of elastic supports and rotor flexibility for dynamics of a cantilever impeller. Journal of Physics: Conference Series, 1741 (1), 012043. doi: https://doi.org/10.1088/1742-6596/1741/1/012043
- Marchenko, A., Tkachuk, M. A., Kravchenko, S., Tkachuk, M. M., Parsadanov, I. (2020). Experimental Tests of Discrete Strengthened Elements of Machine-Building Structures. Advanced Manufacturing Processes, 559–569. doi: https://doi.org/10.1007/978-3-030-40724-7_57
- Subbotina, V., Sobol, O. (2020). Structure and properties of microarc oxide coatings on high-temperature aluminum alloy. Machines. Technologies. Materials, 14 (6), 247–250. Available at: https://stumejournals.com/journals/mtm/2020/6/247
- Subbotinа, V., Sоbоl, O., Belozerov, V., Subbotin, A., Smyrnova, Y. (2020). A study of the phase-structural engineering possibilities of coatings on D16 alloy during micro-arc oxidation in electrolytes of different types. Eastern-European Journal of Enterprise Technologies, 4 (12 (106)), 14–23. doi: https://doi.org/10.15587/1729-4061.2020.209722
- Subbotina, V. V., Al-Qawabeha, U. F., Sobol', O. V., Belozerov, V. V., Schneider, V. V., Tabaza, T. A., Al-Qawabah, S. M. (2019). Increase of the α-Al2O3 phase content in MAO-coating by optimizing the composition of oxidated aluminum alloy. Functional materials, 26 (4), 752–758. doi: https://doi.org/10.15407/fm26.04.752
- Subbotin, O., Bilozerov, V., Volkov, O., Subbotinа, V., Shevtsov, V. (2022). Friction properties of mаo coatings on aluminum alloys. Bulletin of the National Technical University «KhPI» Series: Engineering and CAD, 2, 59–63. doi: https://doi.org/10.20998/2079-0775.2022.2.07
- Asquith, D., Yerokhin, A., James, N., Yates, J., Matthews, A. (2013). Evaluation of Residual Stress Development at the Interface of Plasma Electrolytically Oxidized and Cold-Worked Aluminum. Metallurgical and Materials Transactions A, 44 (10), 4461–4465. doi: https://doi.org/10.1007/s11661-013-1854-0
- Dean, J., Gu, T., Clyne, T. W. (2015). Evaluation of residual stress levels in plasma electrolytic oxidation coatings using a curvature method. Surface and Coatings Technology, 269, 47–53. doi: https://doi.org/10.1016/j.surfcoat.2014.11.006
- Matykina, E., Arrabal, R., Mohedano, M., Mingo, B., Gonzalez, J., Pardo, A., Merino, M. C. (2017). Recent advances in energy efficient PEO processing of aluminium alloys. Transactions of Nonferrous Metals Society of China, 27 (7), 1439–1454. doi: https://doi.org/10.1016/s1003-6326(17)60166-3
- Martin, J., Leone, P., Nominé, A., Veys-Renaux, D., Henrion, G., Belmonte, T. (2015). Influence of electrolyte ageing on the Plasma Electrolytic Oxidation of aluminium. Surface and Coatings Technology, 269, 36–46. doi: https://doi.org/10.1016/j.surfcoat.2014.11.001
- Tkachuk, N. A., Kravchenko, S. A., Pylev, V. A., Parsadanov, I. V., Grabovsky, A. V., Veretelnik, O. V. (2019). Discrete and Continual Strengthening of Contacting Structural Elements: Conception, Mathematical and Numerical Modeling. Nauka i tekhnika, 18 (3), 240–247. URL: https://sat.bntu.by/jour/article/view/1980/1794
- Paggi, M., Barber, J. R. (2011). Contact conductance of rough surfaces composed of modified RMD patches. International Journal of Heat and Mass Transfer, 54 (21-22), 4664–4672. doi: https://doi.org/10.1016/j.ijheatmasstransfer.2011.06.011
- Zavarise, G., Borri-Brunetto, M., Paggi, M. (2007). On the resolution dependence of micromechanical contact models. Wear, 262 (1-2), 42–54. doi: https://doi.org/10.1016/j.wear.2006.03.044
- Pohrt, R., Popov, V. L. (2013). Contact Mechanics of Rough Spheres: Crossover from Fractal to Hertzian Behavior. Advances in Tribology, 2013, 1–4. doi: https://doi.org/10.1155/2013/974178
- Pohrt, R., Popov, V. L. (2013). Contact stiffness of randomly rough surfaces. Scientific Reports, 3 (1). doi: https://doi.org/10.1038/srep03293
- Liu, J., Ke, L., Zhang, C. (2021). Axisymmetric thermoelastic contact of an FGM-coated half-space under a rotating punch. Acta Mechanica, 232 (6), 2361–2378. doi: https://doi.org/10.1007/s00707-021-02940-7
- Liu, T.-J., Yang, F., Yu, H., Aizikovich, S. M. (2019). Axisymmetric adhesive contact problem for functionally graded materials coating based on the linear multi-layered model. Mechanics Based Design of Structures and Machines, 49 (1), 41–58. doi: https://doi.org/10.1080/15397734.2019.1666721
- Martynyak, R. M., Prokopyshyn, I. A., Prokopyshyn, I. I. (2015). Contact of Elastic Bodies with Nonlinear Winkler Surface Layers. Journal of Mathematical Sciences, 205 (4), 535–553. doi: https://doi.org/10.1007/s10958-015-2265-0
- Tkachuk, M. (2018). A numerical method for axisymmetric adhesive contact based on kalker’s variational principle. Eastern-European Journal of Enterprise Technologies, 3 (7 (93)), 34–41. doi: https://doi.org/10.15587/1729-4061.2018.132076
- Tkachuk, M., Grabovskiy, A., Tkachuk, M., Hrechka, I., Sierykov, V. (2021). Contact Interaction of a Ball Piston and a Running Track in a Hydrovolumetric Transmission. Lecture Notes in Mechanical Engineering, 195–203. doi: https://doi.org/10.1007/978-3-030-77823-1_20
- Vollebregt, E. A. H. (2012). 100-fold speed-up of the normal contact problem and other recent developments in «CONTACT». Proceedings of the 9th International Conference on Contact Mechanics and Wear of Rail/Wheel Systems. Chengdu. Available at: https://www.researchgate.net/publication/286332997_100-fold_speed-up_of_the_normal_contact_problem_and_other_recent_developments_in_contact
- Li, J., Berger, E. J. (2003). A semi-analytical approach to three-dimensional normal contact problems with friction. Computational Mechanics, 30 (4), 310–322. doi: https://doi.org/10.1007/s00466-002-0407-y
- Motreanu, D. (2001). Eigenvalue problems for variational-hemivariational inequalities in the sense of P. D. Panagiotopoulos. Nonlinear Analysis, 47 (8), 5101–5112. doi: https://doi.org/10.1016/s0362-546x(01)00620-4
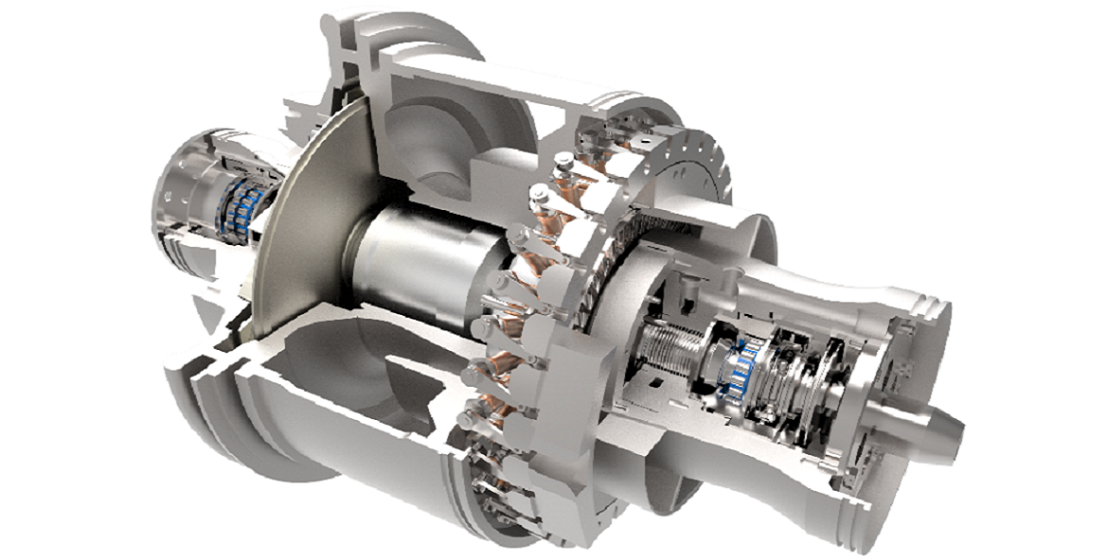
Downloads
Published
How to Cite
Issue
Section
License
Copyright (c) 2023 Mykola Tkachuk, Gennadiy Lvov, Sergey Kravchenko, Serhii Moiseiev, Maksym Novikov, Arkadii Burniashev, Glib Pakki, Serhii Podrieza

This work is licensed under a Creative Commons Attribution 4.0 International License.
The consolidation and conditions for the transfer of copyright (identification of authorship) is carried out in the License Agreement. In particular, the authors reserve the right to the authorship of their manuscript and transfer the first publication of this work to the journal under the terms of the Creative Commons CC BY license. At the same time, they have the right to conclude on their own additional agreements concerning the non-exclusive distribution of the work in the form in which it was published by this journal, but provided that the link to the first publication of the article in this journal is preserved.
A license agreement is a document in which the author warrants that he/she owns all copyright for the work (manuscript, article, etc.).
The authors, signing the License Agreement with TECHNOLOGY CENTER PC, have all rights to the further use of their work, provided that they link to our edition in which the work was published.
According to the terms of the License Agreement, the Publisher TECHNOLOGY CENTER PC does not take away your copyrights and receives permission from the authors to use and dissemination of the publication through the world's scientific resources (own electronic resources, scientometric databases, repositories, libraries, etc.).
In the absence of a signed License Agreement or in the absence of this agreement of identifiers allowing to identify the identity of the author, the editors have no right to work with the manuscript.
It is important to remember that there is another type of agreement between authors and publishers – when copyright is transferred from the authors to the publisher. In this case, the authors lose ownership of their work and may not use it in any way.