Обґрунтування прогресивних технічних рішень турбодетандерних електричних станцій на основі дослідження робочих процесів і станів
DOI:
https://doi.org/10.15587/1729-4061.2023.285865Ключові слова:
дискретно-континуальне зміцнення, контактна взаємодія, напружено-деформований стан, технічна характеристика, турбодетандер, тепломасопереносАнотація
Одним із найбільш раціональних методів утилізації енергії стисненого газу у трубопроводах є турбодетандерні установки. Зокрема, це – автономні турбодетандерні електричні станції. Для підвищення техніко-економічних показників такого типу машин розроблено принципово нову концепцію. Ця концепція орієнтована не на окремий аспект роботи установки, а на весь їх комплекс. Зокрема, як об’єкт досліджень розглядаються фізичні принципи, конструкції та технології. По-перше, на основі моделювання робочих процесів визначено ефективні параметри газодинамічних потоків та тепломасопереносу. По-друге, створені прогресивні конструкції турбодетандерних установок. По-третє, розроблені технології виготовлення деталей та вузлів турбодетандерних установок, що поєднують,на відміну від традиційних, різні види зміцнення для контактуючих деталей у їх парі. Для обґрунтування технічних рішень елементів турбодетандерних електростанцій застосовано метод параметричного моделювання. Це дає можливість визначати технічні характеристики цих установок за певного набору параметрів. Шляхом цілеспрямованого варіювання визначається рекомендований набір їхніх параметрів, які забезпечують підвищення найбільш важливих технічних характеристик. Формується спеціалізована база даних, яка містить масив інформації про закономірності впливу варіювання значущих параметрів на різні характеристики турбодетандерних електростанцій. Уже на цій основі розв’язуються задачі синтезу вдалих технічних рішень турбодетандерних електростанцій. У підсумку забезпечується їх висока енергоефективність. Так, досягається ККД за детандером на рівні 86 % та зростання ресурсу на 20–25 %. Усі ці рішення втілені у низці унікальних турбодетандерних установок. Їх ефективність продемонстрована у ході експлуатації
Посилання
- Avetian, T., Rodriguez, L. (2020). Fundamentals of turboexpander design and operation. Available at: https://fliphtml5.com/gktj/sepi/basic
- Logan Jr., E. (2003). Handbook of Turbomachinery. CRC Press, 880. doi: https://doi.org/10.1201/9780203911990
- Dixon, S. L., Hall, C. A. (2010). Fluid Mechanics and Thermodynamics of Turbomachinery. Elsevier. doi: https://doi.org/10.1016/c2009-0-20205-4
- Simms, J. (2009). Fundamentals of Turboexpanders “basic theory and design”. Available at: https://www.researchgate.net/file.PostFileLoader.html?id=5236f8abd039b1146f66ab16&assetKey=AS%3A272141839208458%401441895078987
- Saravanamuttoo, H. I. H., Cohen, H., Rogers, G. F. C. (2008). Gas Turbine Theory. Pearson. Available at: https://soaneemrana.org/onewebmedia/GAS%20TURBINE%20THEORY%20BY%20HIH%20SARAVANAMUTTOO,%20H.%20COHEN%20&%20GFC%20ROGERS.pdf
- White, F. (2008). Fluid Mechanics. McGraw-Hill. Available at: http://ftp.demec.ufpr.br/disciplinas/TM240/Marchi/Bibliografia/White_2011_7ed_Fluid-Mechanics.pdf
- Kundu, P., Cohen, I., Dowling, D. (2016). Fluid mechanics. Academic Press. doi: https://doi.org/10.1016/c2012-0-00611-4
- Korpela, S. A. (2011). Principles of Turbomachinery. John Wiley & Sons. doi: https://doi.org/10.1002/9781118162477
- Gorla, R. S. R., Khan, A. A. (2003). Turbomachinery Design and Theory. CRC Press. doi: https://doi.org/10.1201/9780203911600
- Expander-generator. Available at: https://turbogaz.com.ua/uk/equipment/dgu_ua
- Moiseev, A. N. (2015). Energoeffektivnaya turboelektromekhanicheskaya sistema dlya gazoraspredelitel'nykh stantsiy. Visnyk NTU «KhPI», 12 (1121), 356–359. Available at: http://library.kpi.kharkov.ua/files/Vestniki/2015_12_0.pdf
- Kuprygin, O., Moiseev, S., Pastukhova, E., Polivanov, V. (2005). Utilizatsionnye turbodetandernye agregaty OAO «Turbogaz». Energetica Moldovei. Aspecte regionale de dezvoltare. Ediția I. Chișinău, 260–265. Available at: https://ibn.idsi.md/vizualizare_articol/64789
- Fluitech Systems. Available at: http://fluitech.com.ua/
- Marchenko, A., Grabovskiy, A., Tkachuk, M., Shut, O., Tkachuk, M. (2021). Detuning of a Supercharger Rotor from Critical Rotational Velocities. Advances in Design, Lecture Notes in Mechanical Engineering, 137–145. doi: https://doi.org/10.1007/978-3-030-77823-1_14
- Tkachuk, M., Shut, O., Marchenko, A., Grabovskiy, A., Lipeiko, A., Polyvianchuk, A. et al. (2021). Strength and Stability Criteria Limiting Geometrical Dimensions of a Cantilever Impeller. SAE Technical Paper Series. doi: https://doi.org/10.4271/2021-01-5056
- Marchenko, A., Pylyov, V., Linkov, O. (2021). Estimation of Strength of the Combustion Chamber of the ICE Piston with a TBC Layer. Integrated Computer Technologies in Mechanical Engineering - 2020, 415–426. doi: https://doi.org/10.1007/978-3-030-66717-7_35
- Tkachuk, M. M., Grabovskiy, A., Tkachuk, M. A., Zarubina, A., Lipeyko, A. (2021). Analysis of elastic supports and rotor flexibility for dynamics of a cantilever impeller. Journal of Physics: Conference Series, 1741 (1), 012043. doi: https://doi.org/10.1088/1742-6596/1741/1/012043
- Marchenko, A., Tkachuk, M. A., Kravchenko, S., Tkachuk, M. M., Parsadanov, I. (2020). Experimental Tests of Discrete Strengthened Elements of Machine-Building Structures. Advanced Manufacturing Processes, 559–569. doi: https://doi.org/10.1007/978-3-030-40724-7_57
- Subbotina, V., Sobol, O. (2020). Structure and properties of microarc oxide coatings on high-temperature aluminum alloy. Machines. Technologies. Materials, 14 (6), 247–250. Available at: https://stumejournals.com/journals/mtm/2020/6/247
- Subbotinа, V., Sоbоl, O., Belozerov, V., Subbotin, A., Smyrnova, Y. (2020). A study of the phase-structural engineering possibilities of coatings on D16 alloy during micro-arc oxidation in electrolytes of different types. Eastern-European Journal of Enterprise Technologies, 4 (12 (106)), 14–23. doi: https://doi.org/10.15587/1729-4061.2020.209722
- Subbotina, V. V., Al-Qawabeha, U. F., Sobol', O. V., Belozerov, V. V., Schneider, V. V., Tabaza, T. A., Al-Qawabah, S. M. (2019). Increase of the α-Al2O3 phase content in MAO-coating by optimizing the composition of oxidated aluminum alloy. Functional materials, 26 (4), 752–758. doi: https://doi.org/10.15407/fm26.04.752
- Subbotin, O., Bilozerov, V., Volkov, O., Subbotinа, V., Shevtsov, V. (2022). Friction properties of mаo coatings on aluminum alloys. Bulletin of the National Technical University «KhPI» Series: Engineering and CAD, 2, 59–63. doi: https://doi.org/10.20998/2079-0775.2022.2.07
- Asquith, D., Yerokhin, A., James, N., Yates, J., Matthews, A. (2013). Evaluation of Residual Stress Development at the Interface of Plasma Electrolytically Oxidized and Cold-Worked Aluminum. Metallurgical and Materials Transactions A, 44 (10), 4461–4465. doi: https://doi.org/10.1007/s11661-013-1854-0
- Dean, J., Gu, T., Clyne, T. W. (2015). Evaluation of residual stress levels in plasma electrolytic oxidation coatings using a curvature method. Surface and Coatings Technology, 269, 47–53. doi: https://doi.org/10.1016/j.surfcoat.2014.11.006
- Matykina, E., Arrabal, R., Mohedano, M., Mingo, B., Gonzalez, J., Pardo, A., Merino, M. C. (2017). Recent advances in energy efficient PEO processing of aluminium alloys. Transactions of Nonferrous Metals Society of China, 27 (7), 1439–1454. doi: https://doi.org/10.1016/s1003-6326(17)60166-3
- Martin, J., Leone, P., Nominé, A., Veys-Renaux, D., Henrion, G., Belmonte, T. (2015). Influence of electrolyte ageing on the Plasma Electrolytic Oxidation of aluminium. Surface and Coatings Technology, 269, 36–46. doi: https://doi.org/10.1016/j.surfcoat.2014.11.001
- Tkachuk, N. A., Kravchenko, S. A., Pylev, V. A., Parsadanov, I. V., Grabovsky, A. V., Veretelnik, O. V. (2019). Discrete and Continual Strengthening of Contacting Structural Elements: Conception, Mathematical and Numerical Modeling. Nauka i tekhnika, 18 (3), 240–247. URL: https://sat.bntu.by/jour/article/view/1980/1794
- Paggi, M., Barber, J. R. (2011). Contact conductance of rough surfaces composed of modified RMD patches. International Journal of Heat and Mass Transfer, 54 (21-22), 4664–4672. doi: https://doi.org/10.1016/j.ijheatmasstransfer.2011.06.011
- Zavarise, G., Borri-Brunetto, M., Paggi, M. (2007). On the resolution dependence of micromechanical contact models. Wear, 262 (1-2), 42–54. doi: https://doi.org/10.1016/j.wear.2006.03.044
- Pohrt, R., Popov, V. L. (2013). Contact Mechanics of Rough Spheres: Crossover from Fractal to Hertzian Behavior. Advances in Tribology, 2013, 1–4. doi: https://doi.org/10.1155/2013/974178
- Pohrt, R., Popov, V. L. (2013). Contact stiffness of randomly rough surfaces. Scientific Reports, 3 (1). doi: https://doi.org/10.1038/srep03293
- Liu, J., Ke, L., Zhang, C. (2021). Axisymmetric thermoelastic contact of an FGM-coated half-space under a rotating punch. Acta Mechanica, 232 (6), 2361–2378. doi: https://doi.org/10.1007/s00707-021-02940-7
- Liu, T.-J., Yang, F., Yu, H., Aizikovich, S. M. (2019). Axisymmetric adhesive contact problem for functionally graded materials coating based on the linear multi-layered model. Mechanics Based Design of Structures and Machines, 49 (1), 41–58. doi: https://doi.org/10.1080/15397734.2019.1666721
- Martynyak, R. M., Prokopyshyn, I. A., Prokopyshyn, I. I. (2015). Contact of Elastic Bodies with Nonlinear Winkler Surface Layers. Journal of Mathematical Sciences, 205 (4), 535–553. doi: https://doi.org/10.1007/s10958-015-2265-0
- Tkachuk, M. (2018). A numerical method for axisymmetric adhesive contact based on kalker’s variational principle. Eastern-European Journal of Enterprise Technologies, 3 (7 (93)), 34–41. doi: https://doi.org/10.15587/1729-4061.2018.132076
- Tkachuk, M., Grabovskiy, A., Tkachuk, M., Hrechka, I., Sierykov, V. (2021). Contact Interaction of a Ball Piston and a Running Track in a Hydrovolumetric Transmission. Lecture Notes in Mechanical Engineering, 195–203. doi: https://doi.org/10.1007/978-3-030-77823-1_20
- Vollebregt, E. A. H. (2012). 100-fold speed-up of the normal contact problem and other recent developments in «CONTACT». Proceedings of the 9th International Conference on Contact Mechanics and Wear of Rail/Wheel Systems. Chengdu. Available at: https://www.researchgate.net/publication/286332997_100-fold_speed-up_of_the_normal_contact_problem_and_other_recent_developments_in_contact
- Li, J., Berger, E. J. (2003). A semi-analytical approach to three-dimensional normal contact problems with friction. Computational Mechanics, 30 (4), 310–322. doi: https://doi.org/10.1007/s00466-002-0407-y
- Motreanu, D. (2001). Eigenvalue problems for variational-hemivariational inequalities in the sense of P. D. Panagiotopoulos. Nonlinear Analysis, 47 (8), 5101–5112. doi: https://doi.org/10.1016/s0362-546x(01)00620-4
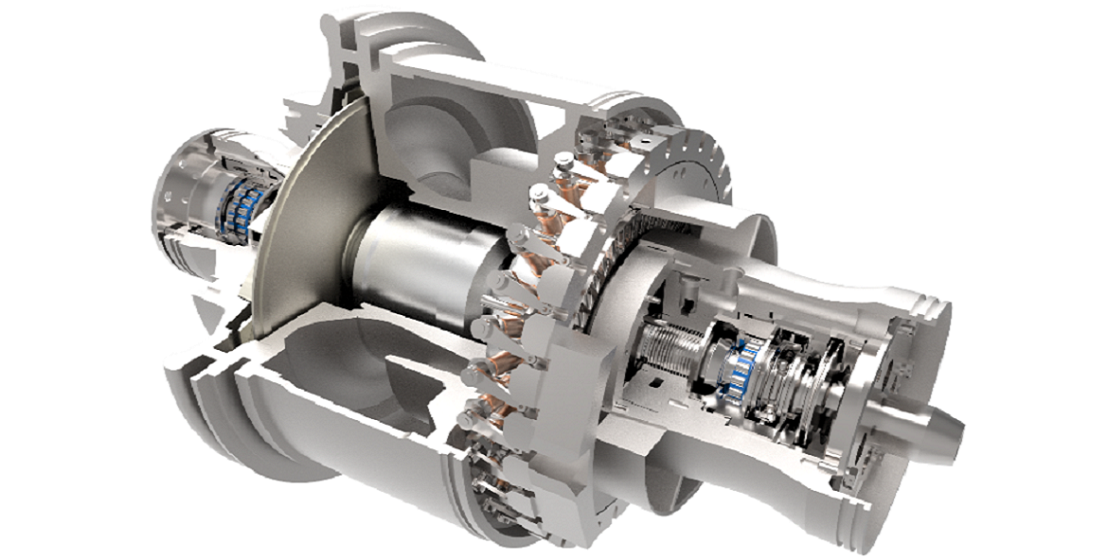
##submission.downloads##
Опубліковано
Як цитувати
Номер
Розділ
Ліцензія
Авторське право (c) 2023 Mykola Tkachuk, Gennadiy Lvov, Sergey Kravchenko, Serhii Moiseiev, Maksym Novikov, Arkadii Burniashev, Glib Pakki, Serhii Podrieza

Ця робота ліцензується відповідно до Creative Commons Attribution 4.0 International License.
Закріплення та умови передачі авторських прав (ідентифікація авторства) здійснюється у Ліцензійному договорі. Зокрема, автори залишають за собою право на авторство свого рукопису та передають журналу право першої публікації цієї роботи на умовах ліцензії Creative Commons CC BY. При цьому вони мають право укладати самостійно додаткові угоди, що стосуються неексклюзивного поширення роботи у тому вигляді, в якому вона була опублікована цим журналом, але за умови збереження посилання на першу публікацію статті в цьому журналі.
Ліцензійний договір – це документ, в якому автор гарантує, що володіє усіма авторськими правами на твір (рукопис, статтю, тощо).
Автори, підписуючи Ліцензійний договір з ПП «ТЕХНОЛОГІЧНИЙ ЦЕНТР», мають усі права на подальше використання свого твору за умови посилання на наше видання, в якому твір опублікований. Відповідно до умов Ліцензійного договору, Видавець ПП «ТЕХНОЛОГІЧНИЙ ЦЕНТР» не забирає ваші авторські права та отримує від авторів дозвіл на використання та розповсюдження публікації через світові наукові ресурси (власні електронні ресурси, наукометричні бази даних, репозитарії, бібліотеки тощо).
За відсутності підписаного Ліцензійного договору або за відсутністю вказаних в цьому договорі ідентифікаторів, що дають змогу ідентифікувати особу автора, редакція не має права працювати з рукописом.
Важливо пам’ятати, що існує і інший тип угоди між авторами та видавцями – коли авторські права передаються від авторів до видавця. В такому разі автори втрачають права власності на свій твір та не можуть його використовувати в будь-який спосіб.