Synthesis of nanocrystalline nickel via pulsed current electrodeposition in additive-free deposition bath and comparison of nanoscale characterization
DOI:
https://doi.org/10.15587/1729-4061.2024.298302Keywords:
nanocrystalline, nickel, nanoscale, electrodeposition, additive, pulsed current, nucleation rate, pyramidal, FWHMAbstract
An experimental investigation on synthesis of nanocrystalline nickels by pulsed current electrodeposition has been carried out in an additive-free Watts bath employing nickel-sulphate solution with similar nickel ion concentrations. Aluminum was used as a substrate. It demonstrated the advantage of easier removal process of electrodeposited nanocrystalline nickel from its substrate. Whereas the use of high-purity nickel anode was intended to replace nickel ions, which decreased during electrodeposition. Different peak current densities of 450, 750 and 1000 mA/cm2 were applied. A pulsed current was set at a similar pulse pattern of on-time and off-time of 1 ms and 9 ms respectively. The shorter on-time demonstrated the ability to limit ion deposition, which was related to the formation of finer grains. The off-time arrangement was targeted to ensure that the ion mobility had completely stopped. Higher current density demonstrated a dominant impact on deposits, generating a higher nucleation rate that is related to depositing nanocrystalline nickel. A peak current density of 1000 mA/cm2 produced grain sizes in the nanoscale regime.
Without any additional additive, nanocrystalline nickel was successfully yielded. Investigation of grain size obtained from the 1000 mA/cm2 has been conducted by extracting full width at half maximum peak intensity (FWHM) revealed from X-ray diffraction (XRD) and Transmission Electron Microscopy (TEM) exhibited consistent results of 22 nm and 25.4±3.4 nm, respectively. It is also evidence of the significant role of pulsed current density. In inclusion, nanocrystalline nickel can be synthesized in an electrodeposition bath without any addition of additives
References
- Wasekar, N. P., Haridoss, P., Seshadri, S. K., Sundararajan, G. (2016). Influence of mode of electrodeposition, current density and saccharin on the microstructure and hardness of electrodeposited nanocrystalline nickel coatings. Surface and Coatings Technology, 291, 130–140. https://doi.org/10.1016/j.surfcoat.2016.02.024
- Dong, Y., Yang, H., Zhang, L., Li, X., Ding, D., Wang, X. et al. (2020). Ultra‐Uniform Nanocrystalline Materials via Two‐Step Sintering. Advanced Functional Materials, 31 (1). https://doi.org/10.1002/adfm.202007750
- Zhang, F., Yao, Z., Moliar, O., Tao, X., Yang, C. (2020). Nanocrystalline Ni coating prepared by a novel electrodeposition. Journal of Alloys and Compounds, 830, 153785. https://doi.org/10.1016/j.jallcom.2020.153785
- Klapper, H. S., Zadorozne, N. S., Rebak, R. B. (2017). Localized Corrosion Characteristics of Nickel Alloys: A Review. Acta Metallurgica Sinica (English Letters), 30 (4), 296–305. https://doi.org/10.1007/s40195-017-0553-z
- Wang, F., Li, L., Liu, J., Shu, Q. (2017). Research on tool wear of milling nickel-based superalloy in cryogenic. The International Journal of Advanced Manufacturing Technology, 91 (9-12), 3877–3886. https://doi.org/10.1007/s00170-017-0079-6
- Zhou, X., Ouyang, C. (2017). Anodized porous titanium coated with Ni-CeO2 deposits for enhancing surface toughness and wear resistance. Applied Surface Science, 405, 476–488. https://doi.org/10.1016/j.apsusc.2017.02.034
- Merita, F., Umemoto, D., Yuasa, M., Miyamoto, H., Goto, T. (2018). Electrodeposition of nanocrystalline nickel embedded with inert nanoparticles formed via inverse hydrolysis. Applied Surface Science, 458, 612–618. https://doi.org/10.1016/j.apsusc.2018.07.123
- Gu, C., Lian, J., He, J., Jiang, Z., Jiang, Q. (2006). High corrosion-resistance nanocrystalline Ni coating on AZ91D magnesium alloy. Surface and Coatings Technology, 200 (18-19), 5413–5418. https://doi.org/10.1016/j.surfcoat.2005.07.001
- Zhou, X., Wu, F., Ouyang, C. (2017). Electroless Ni–P alloys on nanoporous ATO surface of Ti substrate. Journal of Materials Science, 53 (4), 2812–2829. https://doi.org/10.1007/s10853-017-1686-1
- Fratesi, R., Ruffini, N., Malavolta, M., Bellezze, T. (2002). Contemporary use of Ni and Bi in hot-dip galvanizing. Surface and Coatings Technology, 157 (1), 34–39. https://doi.org/10.1016/s0257-8972(02)00137-8
- Zeng, C., Tian, W., Liao, W. H., Hua, L. (2016). Microstructure and porosity evaluation in laser-cladding deposited Ni-based coatings. Surface and Coatings Technology, 294, 122–130. https://doi.org/10.1016/j.surfcoat.2016.03.083
- Sadeghimeresht, E., Markocsan, N., Nylén, P., Björklund, S. (2016). Corrosion performance of bi-layer Ni/Cr 2 C 3 –NiCr HVAF thermal spray coating. Applied Surface Science, 369, 470–481. https://doi.org/10.1016/j.apsusc.2016.02.002
- John, A., Saeed, A., Khan, Z. A. (2023). Influence of the Duty Cycle of Pulse Electrodeposition-Coated Ni-Al2O3 Nanocomposites on Surface Roughness Properties. Materials, 16 (6), 2192. https://doi.org/10.3390/ma16062192
- Matsui, I., Watanabe, A., Takigawa, Y., Omura, N., Yamamoto, T. (2020). Microstructural heterogeneity in the electrodeposited Ni: insights from growth modes. Scientific Reports, 10 (1). https://doi.org/10.1038/s41598-020-62565-z
- Watanabe, A., Yamamoto, T., Takigawa, Y. (2022). Tensile strength of nanocrystalline FeCoNi medium-entropy alloy fabricated using electrodeposition. Scientific Reports, 12 (1). https://doi.org/10.1038/s41598-022-16086-6
- Cheng, A.-Y., Pu, N.-W., Liu, Y.-M., Hsieh, M.-S., Ger, M.-D. (2023). Evaluation of Ni–B alloy electroplated with different anionic groups. Journal of Materials Research and Technology, 27, 8360–8371. https://doi.org/10.1016/j.jmrt.2023.11.243
- Rashidi, A. M., Amadeh, A. (2008). The effect of current density on the grain size of electrodeposited nanocrystalline nickel coatings. Surface and Coatings Technology, 202 (16), 3772–3776. https://doi.org/10.1016/j.surfcoat.2008.01.018
- Moti, E., Shariat, M. H., Bahrololoom, M. E. (2008). Electrodeposition of nanocrystalline nickel by using rotating cylindrical electrodes. Materials Chemistry and Physics, 111 (2-3), 469–474. https://doi.org/10.1016/j.matchemphys.2008.04.051
- Liu, H., Wang, H., Ying, W., Liu, W., Wang, Y., Li, Q. (2020). Influences of Duty Cycle and Pulse Frequency on Properties of Ni-SiC Nanocomposites fabricated by Pulse Electrodeposition. International Journal of Electrochemical Science, 15 (10), 10550–10569. https://doi.org/10.20964/2020.10.33
- Lv, B., Hu, Z., Wang, X., Xu, B. (2015). Electrodeposition of nanocrystalline nickel assisted by flexible friction from an additive-free Watts bath. Surface and Coatings Technology, 270, 123–131. https://doi.org/10.1016/j.surfcoat.2015.03.012
- Nayana, K. O., Ranganatha, S., Shubha, H. N., Pandurangappa, M. (2019). Effect of sodium lauryl sulphate on microstructure, corrosion resistance and microhardness of electrodeposition of Ni–Co3O4 composite coatings. Transactions of Nonferrous Metals Society of China, 29 (11), 2371–2383. https://doi.org/10.1016/s1003-6326(19)65143-5
- Chauhan, M., Mohamed, F. A. (2006). Investigation of low temperature thermal stability in bulk nanocrystalline Ni. Materials Science and Engineering: A, 427 (1-2), 7–15. https://doi.org/10.1016/j.msea.2005.10.039
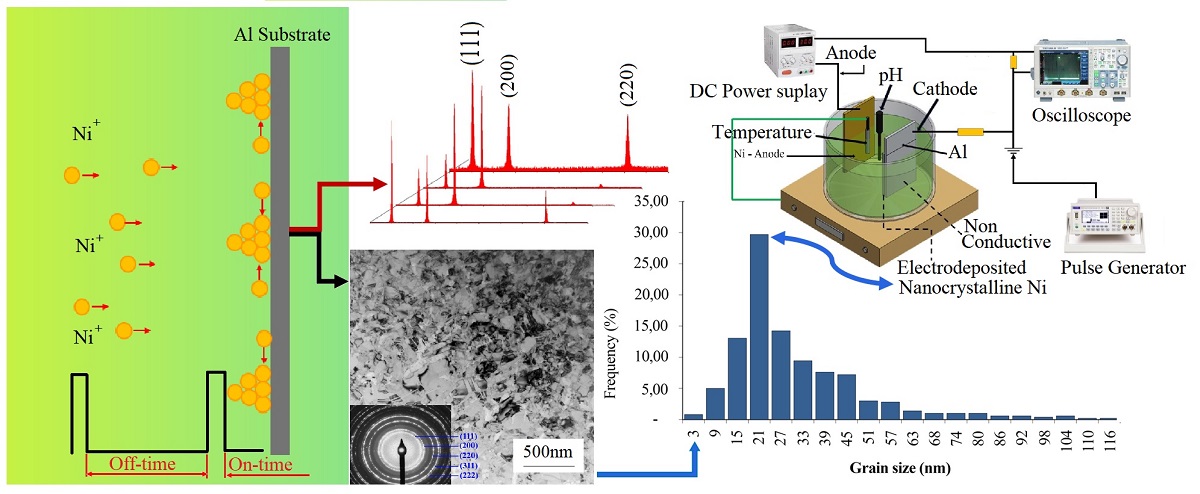
Downloads
Published
How to Cite
Issue
Section
License
Copyright (c) 2024 Rahmad Imbang Tritjahjono

This work is licensed under a Creative Commons Attribution 4.0 International License.
The consolidation and conditions for the transfer of copyright (identification of authorship) is carried out in the License Agreement. In particular, the authors reserve the right to the authorship of their manuscript and transfer the first publication of this work to the journal under the terms of the Creative Commons CC BY license. At the same time, they have the right to conclude on their own additional agreements concerning the non-exclusive distribution of the work in the form in which it was published by this journal, but provided that the link to the first publication of the article in this journal is preserved.
A license agreement is a document in which the author warrants that he/she owns all copyright for the work (manuscript, article, etc.).
The authors, signing the License Agreement with TECHNOLOGY CENTER PC, have all rights to the further use of their work, provided that they link to our edition in which the work was published.
According to the terms of the License Agreement, the Publisher TECHNOLOGY CENTER PC does not take away your copyrights and receives permission from the authors to use and dissemination of the publication through the world's scientific resources (own electronic resources, scientometric databases, repositories, libraries, etc.).
In the absence of a signed License Agreement or in the absence of this agreement of identifiers allowing to identify the identity of the author, the editors have no right to work with the manuscript.
It is important to remember that there is another type of agreement between authors and publishers – when copyright is transferred from the authors to the publisher. In this case, the authors lose ownership of their work and may not use it in any way.